Cutting-Edge Lithium-Ion Battery Development is Supported by Thermal Analysis Research
Highlights from the world’s leading lithium-ion battery development labs using thermal analysis to create safer and better battery materials
Morgan Ulrich | Chris Stumpf
March 21, 2022
Whether you’ve used a cell phone or driven an electric vehicle (please, not at the same time), you’ve probably come to realize that lithium-ion batteries are taking over the energy world. They power our portable electronics, vital medical equipment, electric vehicles, and renewable energy storage. As the market expands, researchers are finding ways to make Li-ion batteries increasingly powerful, dependable, and safe, all while minimizing production time and cost.
As new batteries are developed, safety remains a top concern since Li-ion batteries have a tendency to overheat – through thermal, electric, and mechanical abuses – in some cases leading to thermal runaway and combustion.
Thermal analysis techniques elucidate how battery materials respond to thermal stresses, enabling battery scientists to create safer and better performing batteries. A battery thermal management system is just one example of where thermal property information gained from thermal analysis can help produce safer batteries by ensuring that operating temperatures stay below the point at which a battery material may start to degrade.
The following research examples highlight how the world’s leading Li-ion battery development labs use the thermal analysis techniques of TGA, DSC, and TGA-MS to support innovative product design and testing.
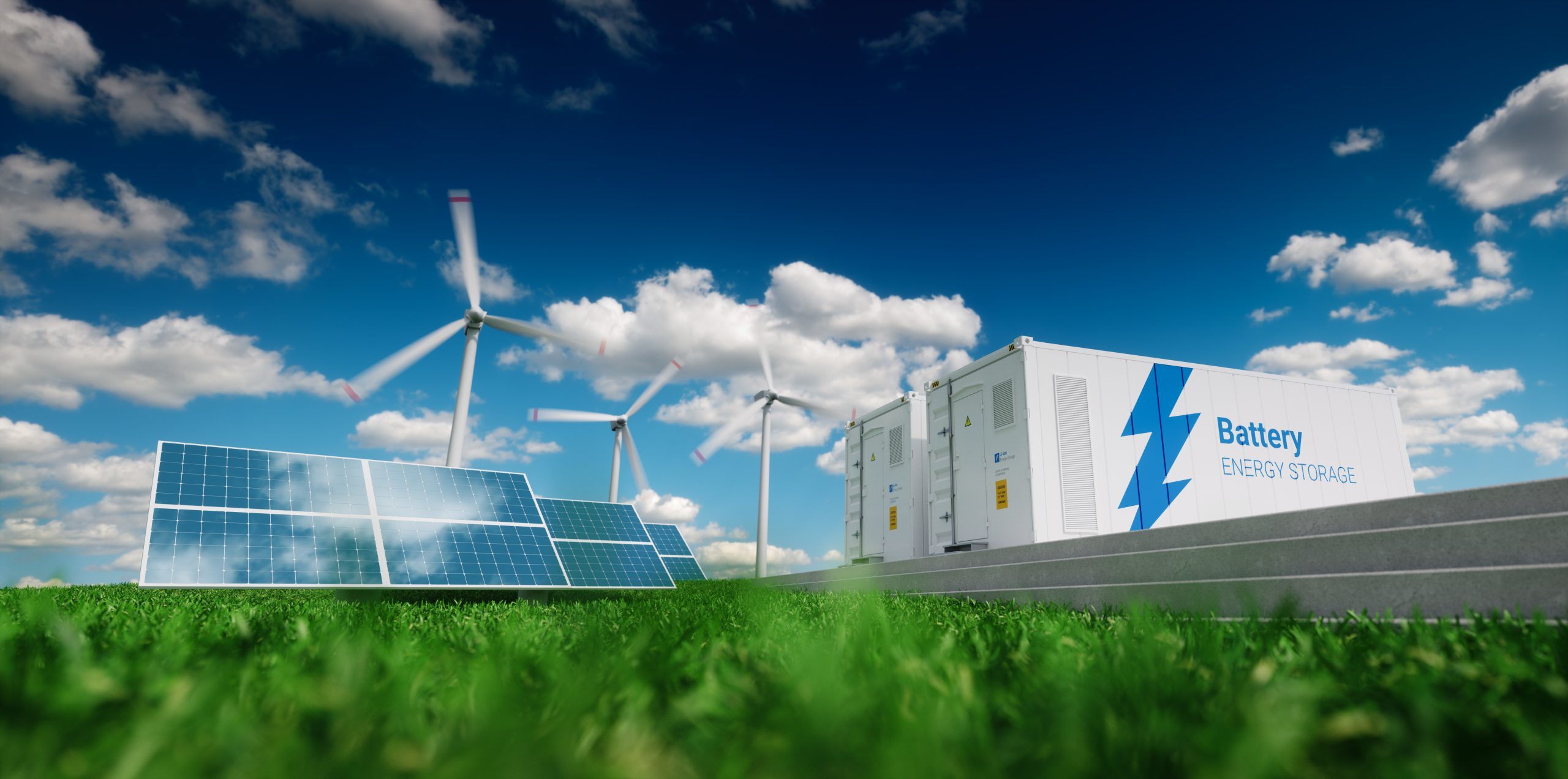
Differential Scanning Calorimetry (DSC) for advancing our understanding of thermal runaway in Li-ion materials
Differential Scanning Calorimeters (DSC) measure the heat absorbed or released when a sample material is heated, cooled, or held isothermal. The heat flow is determined by comparing the heat flow difference between a sample material and a reference. DSC provides insights into the battery material’s heat capacity and phase transitions such as melting point (Tm), heat of fusion, and glass transition (Tg).
Li-ion batteries generally operate within a temperature range of -20 to 60 °C, but mechanical, electric, or thermal abuses can lead to an excessive rise and fall of temperature within a battery that can result in a cataclysmic event called thermal runaway. The exact nature of the thermodynamic and kinetic mechanisms of thermal runaway are still an area of active research. Studies indicate that the initial step of thermal runaway may begin with decomposition of the solid electrolyte interface (SEI) at around 80 to 120 °C. At progressively higher temperatures, other materials within the battery begin to decompose and interact.
An innovative study by Zhou et. al. from Purdue University leveraged a TA Instruments DSC to build a computational model for understanding and predicting thermal runaway. Their study proves that interactions between the battery electrodes trigger the catastrophic safety event at the single-cell level. Their insightful research moves us one step closer to understanding the mechanisms of thermal runaway and gives us a better understanding of battery safety.
Thermogravimetric Analysis (TGA) used to find Li-ion battery materials capable of operating at higher temperatures
Thermogravimetric Analyzers (TGA) programmatically heat up a material while measuring its mass change with a highly sensitive analytical balance. Loss of mass indicates possible decomposition or vaporization, while a gain in mass represents possible sorption or that the material is reacting with its gaseous surroundings. Battery developers turn to TGA to quantify oxidation, thermal degradation, and thermal stability. TGA elucidates the temperatures at which battery materials start to degrade, empowering researchers to choose proper materials and build high performing, long-lasting batteries.
Commercially available lithium-ion batteries utilize organic based carbonate solvents. One downside of these organic carbonate solvents is that at elevated temperatures they may be flammable. An alternative to organic carbonate solvents are polymer and gel-based electrolytes. Cresce et al used a TA Instruments TGA to test their manufacturing-friendly acrylate gel electrolyte system in an aqueous-based lithium-ion battery. They discovered that their gel changes composition above 90 °C. Therefore, their battery is favorable over state-of-the-art organic electrolyte batteries using LiPF6 salt, which incur degradation at just 70 °C. The Cresce research team benefitted from TGA’s exact measurements of gel electrolytes’ weight change at different temperatures, enabling confident conclusions about the safety of their designs.
Kohlmeyer et al took a different approach and designed a new electrolyte and separator combination for high temperature battery operation. They studied their membranes’ thermal stability on a TA Instruments TGA. The LiFePO4//graphite with their membrane and electrolytes system were shown to operate at 120 °C with excellent cyclability which is significantly above the operation temperature of traditional Li-ion battery. Their breakthrough paves the way for a future of Li-ion batteries capable of operating safely at higher temperatures than ever before.
Thermogravimetric Analysis / Evolved Gas Analysis (TGA-EGA) used to develop Li-salts with reduced evolved hydrofluoric acid
The examples above demonstrate how DSC and TGA can be used to measure thermal profiles of battery materials, thereby greatly contributing to improved battery materials and safety. Scientists can also employ these methods in tandem with the complementary technique of evolved gas analysis (EGA). Battery researchers can use EGA to understand the gases that are being evolved during a thermal measurement on a TGA.
TGA-MS is a technique that combines thermogravimetric analysis (TGA) and mass spectrometry (MS) yielding thermal stability data and insight into the chemical composition of the evolved gases (it is also possible to hyphenate GC/MS and FT-IR to TGA). One advantage is that there is no additional sample preparation required than that required for a simple TGA experiment.
Traditional Li-salts, such as LiPF6, can emit toxic and unsafe gaseous products during thermal degradation, e.g., hydrofluoric acid (HF). Alternatives to the traditional Li-salts are an active area of research to make safer Li-ion batteries. Paillet et. al. demonstrated the power of their TA Instruments’ TGA-MS in characterizing lithium 4,5-dicyano-2-(trifluoromethyl) imidazolide (LiTDI) salt for lithium ion batteries. The LiTDI salt was compared to commonly used LiPF6. The Paillet study showed that the thermal stability of LiTDI versus LiPF6 was dramatically better for LiTDI (285 °C versus 164 °C). The authors also showed that LiTDI was safer with regards to HF gas evolution. They accomplished this by plotting m/z 19 as a function of temperature from their TGA-MS study. The LiTDI showed less evolution of HF relative to LiPF6 while at the same time demonstrating a similar power capability to LiPF6, representing a promising electrolyte for future Li-ion batteries with significant improvement in safety.
Thermal Analysis Enabled Their Discoveries – What Next?
DSC, TGA, and TGA-MS played critical roles in the discoveries mentioned here. From detailed battery material analysis to whole-battery performance, these techniques enabled researchers to determine which designs were safe and effective under specific conditions. They all aimed to push the capabilities of Li-ion batteries further to enhance performance at high temperatures, which is a trend that will surely continue as Li-ion batteries are increasingly used in everyday devices and applications.
*Note: Some of the instruments used in these references use previous generation models. The instruments linked in this article highlight the current generation models with detailed information about their testing capabilities.
References:
- Cresce, A., Eidson, N., Schroeder, M., Ma, L., Howarth, Y., Yang, C., Ho, J., Dillon, R., Ding, M., Bassett, A. (2020). Gel electrolyte for a 4V flexible aqueous lithium-ion battery.” Journal of Power Sources, 469. https://doi.org/10.1016/j.jpowsour.2020.228378
- Kohlmeyer, R. R., Horrocks, G. A., Blake, A. J., Yu, Z., Maruyama, B., Huang, H., Durstock, M. F. (2020). Pushing the thermal limits of Li-ion batteries. Nano Energy, 64. https://doi.org/10.1016/j.nanoen.2019.103927
- Paillet, S., Schmidt, G., Ladouceur, S., Frechette, J., Barray, F., Clement, D., Hovington, P., Guerfi, A., Vijh, A., Cayrefourcq, I., Zaghib, K. Power capability of LiTDI-based electrolytes for lithium-ion batteries (2015). Journal of Power Sources, 294. http://dx.doi.org/10.1016/j.jpowsour.2015.06.073
- Zhou, H., Mukul Parmananda, M., Crompton, M. K., Hladky, M. P., Dann, M. A., Ostanek, J. A., Mukherjee, P. P. (2022). Effect of electrode crosstalk on heat release in lithium-ion batteries under thermal abuse scenarios. Energy Storage Materials, 44, 326-341. https://doi.org/10.1016/j.ensm.2021.10.030
Other Resources
- Webinar – Improving Li-ion Battery Technology through Advanced Material Analysis
- Webinar – Unlock a New Dimension in your Battery Research Through Isothermal Microcalorimetry
- Webinar – Applications for Isothermal Heat Flow Calorimetry – Lithium Ion Battery Chemistry
- Webinar – Enhanced Understanding of Lithium ion Battery Chemistry Through Isothermal Calorimetry
- Application Note – The Impact of Electrolyte Additives in Lithium-ion Batteries Determined Using Isothermal Microcalorimetry
- Application Note – Microcalorimetry for studying the electrolyte stability of lithium/manganese dioxide batteries