Keywords: β-relaxation, poly(ethyl methacrylate), graphene nanocomposites, rheometer
RH121
Abstract
The β-relaxation of poly (n-alkyl methacrylates) has been a research topic of interest for many years and it is important to study the shift and extent of the β-relaxation to understand its influence on mechanical properties. There has been much recent work to study the glass transition temperature change for graphene polymer nanocomposites such as graphene/PMMA. However, the influence of graphene on the β-relaxation of poly (n-alkyl methacrylates) has not been explored. Here we use an ARES Rheometer with a rectangular torsion fixture, to investigate the β-relaxation change of poly (ethylmethacrylate)/graphene oxide nanocomposites. The dynamic temperature ramp methodology ranged from -40 °C and 60 °C. Compared to the parallel plate experiment, rectangular torsion fixtures can avoid the slip of sample from the plates at low temperatures and reduce sample stiffness to give more accurate results. It was found that 0.12 v% graphene oxide can increase the β-relaxation temperature by 6 K but does not change the intensity and breadth of the β-relaxation. Interestingly, a 15 K increase in the glass transition temperature was also observed, which implies that graphene oxide can split glass transition and β-relaxations.
Introduction
For solid polymers, the mobility of the main chain and side groups is important to determine the mechanical properties. The glass transition, related to the segmental motion of the polymer chains, has attracted vast research interest because of the accompanying significant mechanical property changes. However, the β- relaxation, which is the first relaxation below the glass transition temperature, is also important. It relates specific molecular motions to the viscoelastic response. For example, the β-relaxation has been related to the side group motion of poly (n-alkyl methacrylates).1 It also has been related to cooperative motion of some repeating units along polycarbonate backbone chains.2 The β-relaxation is important for a material’s stiffness, toughness3 and nonlinear response.4
Dispersion of reinforcing inorganic nanoparticles into polymer matrices has been a major strategy to enhance material properties. Interestingly, addition of nanoparticles may also change the glass transition and β-relaxation because of the high surface area available to interact with the polymer chains. Recently, two-dimensional nanofillers of single layer graphite,6,7 such as graphene, and graphene oxide, have aroused significant research interest.8,9,10,11 However, most studies of graphene polymer nanocomposites have only reported that graphene can raise the glass transition temperature Tg of the polymer matrix, such as PMMA with graphene oxide.8,9 The influence of graphene on the β-relaxation of the polymer matrices has been little explored.
Here we describe the application of an ARES Rheometer (TA Instruments) with a rectangular torsion fixture, to investigate the β-relaxation change of poly (ethyl methacrylate)/ graphene oxide. It is observed that graphene increases both the glass transition and the β-relaxation temperature, but the β-relaxation to a lesser extent.
Experiments
Frequently, parallel plate fixtures are used in rheometry of polymers. However, there are two problems accompanying the use of the parallel plate fixtures in the ARES Rheometer in the study of the β-relaxation of solid polymers: slip and instrument compliance. Here we use a rectangular torsion fixture to solve these problems.(See Figure 1)
When below Tg, the sample is in the glassy regime where the modulus is high hence a large torque can be generated when a small shear strain is applied to the sample. Consequently, the sample can slip from the surface of the plates, leading to error in the results, or even inability to perform the test. However, rectangular torsion fixtures hold the sample using clamps, hence avoiding slip.
Instrument compliance is also a problem that can lead to incorrect results, especially when the sample is stiff when close to and below Tg.12 The sample using parallel plates is short with a relatively large diameter. For example, a cylindrical sample with the diameter of 8 mm and 1 mm height might be used. Using this configuration, the stiffness of the sample can be large enough to approach the instrument stiffness, which results in a considerable displacement of the instrument itself, rather than the sample. Since the measured compliance is the sum of the compliances of instrument and sample, in this case the apparent sample stiffness reported by the instrument is much lower than the actual value.
For example, using 6 mm diameter parallel plates, Shi et al13 reported a 450 MPa glassy modulus for glycerol, but when considering the instrument compliance issue, the corrected glass modulus is 3.5 GPa.14
To solve the instrument compliance problem, here we use a rectangular torsion fixture and the sample is a thin rectangular sheet such as 1.3 × 8.0 × 30 mm3. The sample with this geometry has a much lower stiffness compared to the instrument itself. Therefore the displacement of the instrument itself is very small and can either be neglected or a small correction can be made.
To study the β-relaxation of PEMA/graphene oxide, dynamic temperature ramps were run from -40 to 60 °C at 1Hz (2π rad/s) frequency, 0.02 % strain and a heating rate of 1°C / min, in auto-tension mode. The sample was prepared as described previously11 and molded through compression molding using a hot-press at 473 K for 5 mins.
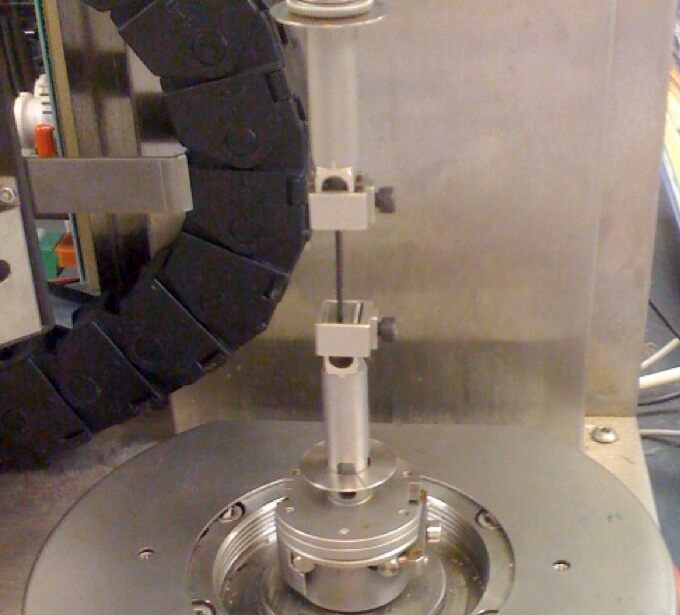
Results
The β-relaxation can be located by the temperature at which there is a loss modulus G”(w) peak. It was found that 0.12 v% graphene oxide can increase the β-relaxation temperature by 6 K but doesn’t change the intensity of β- relaxation peak. (Figure 2) This indicates that graphene oxide does not reinforce PEMA by suppressing the β-relaxation as would be the case for an anti-plastizer.15
Interestingly, Q20 DSC gives a 15 K increase of the glass transition temperature of PEMA.(Figure 3) The incorporation of graphene oxide splits the glass transition and β-relaxation of poly (ethyl methacrylate), similar to results reported for an epoxy/ POSS nanocomposite system.16
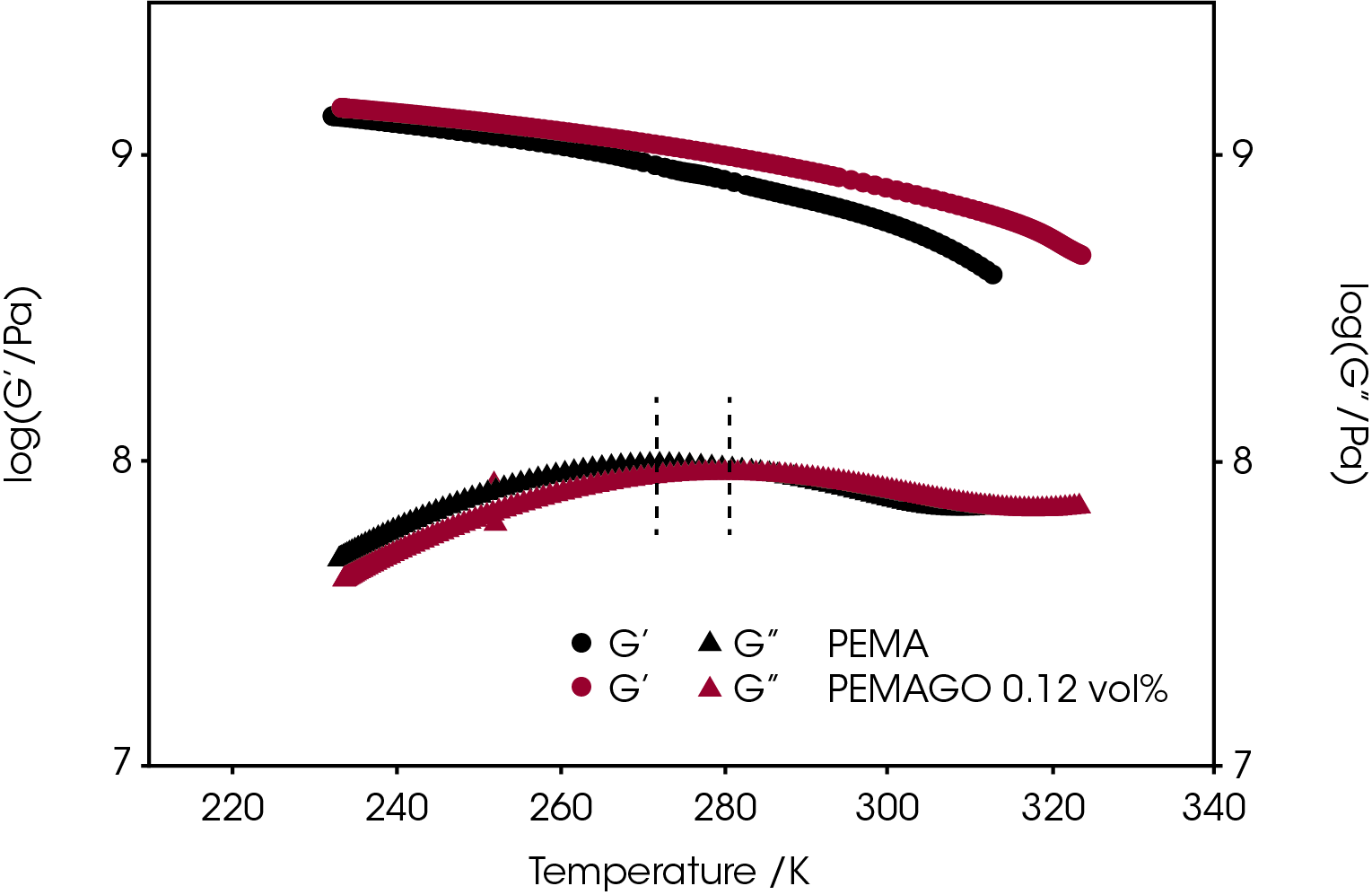
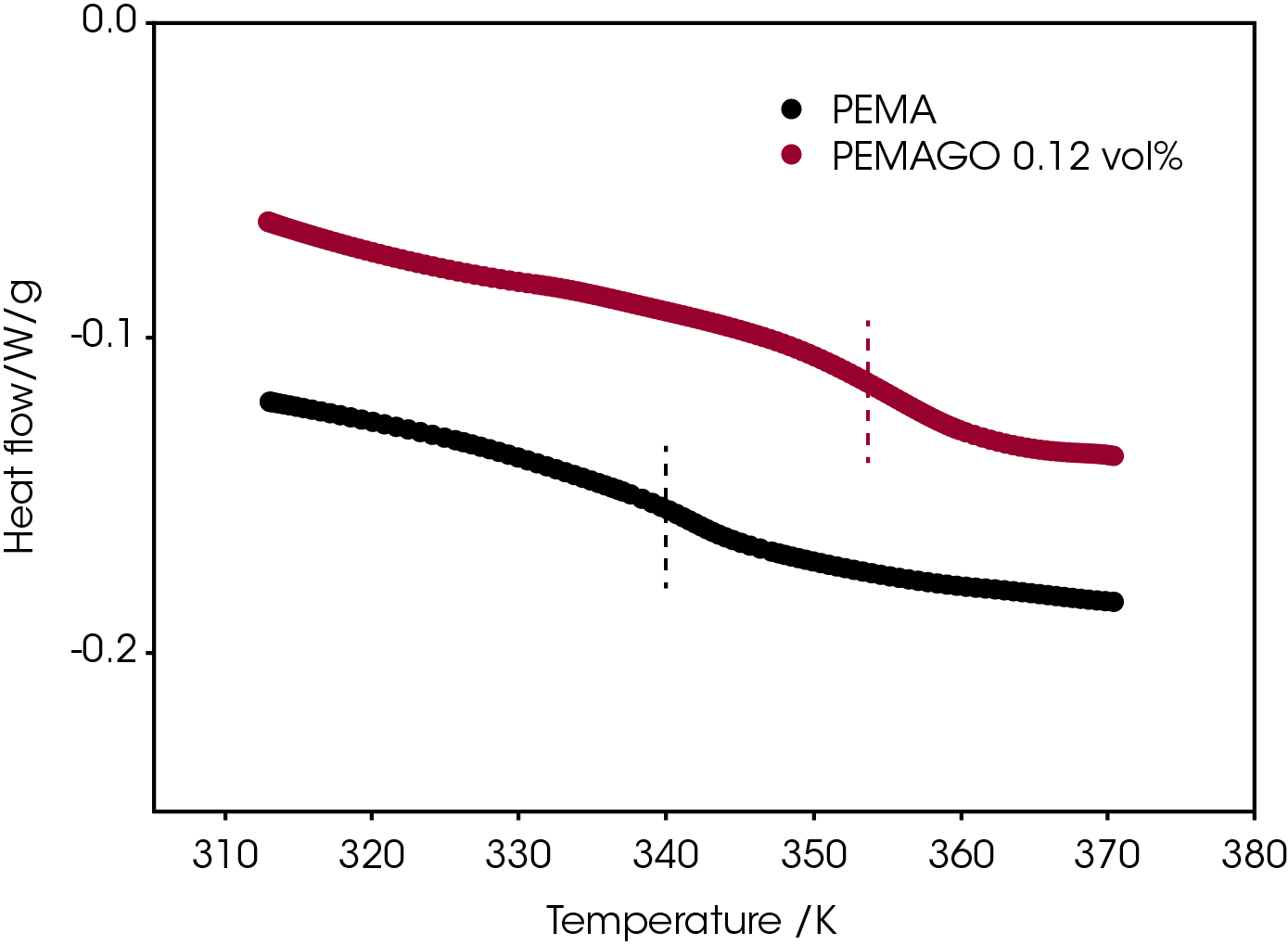
Conclusion
An ARES rheometer with a rectangular torsion fixture was used to study the β-relaxation change of a poly (ethyl methacrylate) upon the addition of graphene oxide. The incorporation of 0.12 vol % of graphene oxide does not suppress the β-relaxation and it can shift both β-relaxation temperature and Tg to higher temperatures. We also report an increase in the distance between β-relaxation and glass transition.
References
- J. Heijboer In Physics of Non-crystalline Solids; Prins, E. B.J. A., Ed.; Wiley: New York, 1965.
- A.F. Yee and S.A. Smith, Macromolecules 1981, 14, 54.
- R. F. Boyer, Polymer Engineering & Science, 1968, 8, 161.
- A. Flory and G. B. McKenna, Macromolecules 2005, 38, 1760
- S. Cervenya, S.N. Goyanesa, A.J. Marzoccaa and G.H. Rubioloa, Polymer , 1999, 40, 1495
- K. Geim and K.S. Novoselov, Nat. Mater, 2007, 6, 183
- H. Kim, A.A Abdala and C.W. Macosko, Macromolecules, 2010, 43, 6515
- T. Ramanathan, A.A Abdala, S. Stankovich, D.A. Dikin, M. Herrera-Alonso, R.D. Piner, D.H. Adamson, H.C. Schniepp, X. Chen, R.S. Ruoff, S.T. Nguyen, I.A. Aksay, R.K. Prud’Homme and L.C. Brinson, Nature Nanotechnol, 3 RS051 2008, 3, 327 (2008).
- J. R. Potts, S. H. Lee, T. M. Alam, J. An, M. D. Stoller, R. D. Piner and R.S. Ruoff, Carbon, 2011, 49, 2615
- X. Li, G.B. McKenna, G. Miquelard-Garnier, A. Guinault, C. Sollogoub, G. Regnier and A. Rozanski. Polymer, 2013, submitted.
- X. Li, and G.B. McKenna, ACS Macro Letters, 2012, 1, 388
- S. A. Hutcheson and G. B. McKenna, J. Chem. Phys, 2008, 129, 074502
- X. Shi, A. Mandanici and G. B. McKenna, J. Chem. Phys, 2005, 123, 174507
- K. Schröter , S. A. Hutcheson , X. Shi, A. Mandanici and G. B. McKenna, J. Chem. Phys, 2006, 125, 2145907
- L.M. Robeson, and J.A. Faucher, Polym. Lett. 1969, 7, 35.
- Q.Li, S.A. Hutcheson, G.B. McKenna and S.L. Simon, J Polym Sci Part B: Poly Phys, 2008, 46, 2719
Acknowledgement
This work was contributed by Xiguang Li, under the supervision of Gregory B. McKenna in the Department of Chemical Engineering at Texas Tech University. The authors acknowledge the John R. Bradford Endowment at Texas Tech University and the National Science Foundation under grant CHE-1112416 both for partial support of this work.
Click here to download the printable version of this application note.