Keywords: Melting Temperature, Heat of Fusion, Apparent Melting, Thermodynamic Melting, Kinetic Process, Differential Scanning Calorimetry, Decomposition, Drug-Excipient Interaction
TA401
Abstract
Differential Scanning Calorimetry (DSC) is an analytical technique frequently used to detect and quantify crystalline content of pharmaceuticals, foods, polymers and many other materials. The presence of crystalline structure is seen as an endothermic peak during heating in a DSC experiment. The temperature and area of the peak can be used to help identify, qualify, and quantify crystalline structure. Most DSC users and materials researchers typically know or suspect if their material contains crystalline content. Most commonly, a DSC thermogram containing an endothermic peak is often interpreted as “thermodynamic melting” of crystalline structure. However, this interpretation of the data may not always be correct. For example, numerous papers1,2,3 have been published in the last few years to illustrate how the start of chemical reactions (hydrolysis) and thermal decomposition in many common sugars, such as sucrose, cause loss of crystalline structure as much as 70 °C below typically reported melting temperatures. However, some researchers remain skeptical of the interpretation of these findings.
The purpose of this paper is to provide a universally acceptable definition of “Melting” and then illustrate that loss of crystalline structure in many materials can be caused by numerous chemical processes other than thermodynamic or “True Melting”. Loss of crystalline structure caused by a chemical process will be called “Apparent Melting” in this paper.
Some may think that since crystalline structure is lost by both True and Apparent melting, it makes no difference in the usefulness and application of the DSC data. That is an incorrect assumption as will be discussed and illustrated. The most important point of this paper is to show how the accuracy (melting temperature and heat of fusion) and utility of the data is greatly diminished for materials that undergo Apparent Melting.
Introduction
Melting of substances is a commonly observed phase change from a crystalline solid to an amorphous liquid. There is a typical assumption that loss of crystalline structure while heating in a DSC at typical rates of 1 to 25 °C/min is True (thermodynamic) Melting. As will be shown, loss of crystalline structure can be caused by numerous processes (kinetic) other than thermodynamic melting. The only common characteristic between True and Apparent melting is conversion from crystalline to amorphous structure.
Although others may have suggested parts of a definition prior to Wunderlich4, his seems to be the most complete. His definition states that thermodynamic melting occurs:
- 1. At a single, time-independent (and therefore heating rate independent) temperature where the corresponding crystalline solid and liquid amorphous phase are in thermal equilibrium (ΔG = 0) at constant pressure
- Without chemical change
An example of Apparent Melting is seen in Figure 1. Most DSC users would assume that the peak is due to True Melting. As will be shown, it takes only two DSC experiments to distinguish between True and Apparent Melting.
If a researcher is unfamiliar with Acetylsalicylic Acid, they will likely do a literature search to find information on the melting point as shown in Figure 2.

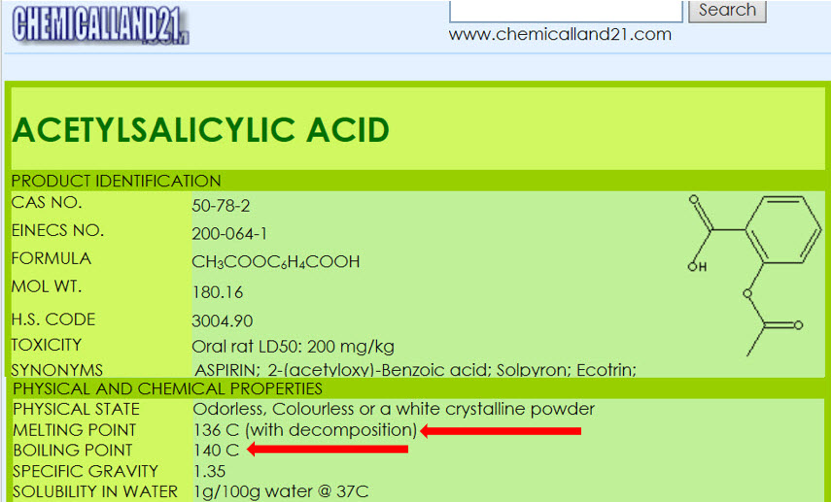
A common term used in the literature for materials that decompose prior to or during melting is “melts with decomposition”. If the term “melts” is an accurate description for loss of crystalline structure then the onset temperature of the endothermic peak would remain constant with heating rate because, as stated in the definition, thermodynamic melting occurs at a single, time independent temperature. Conversely, decomposition is a kinetic process that is time dependent (heating rate dependent). If loss of crystalline structure is due to the onset of thermal decomposition then the onset temperature will shift to higher temperatures at higher heating rates. The series of heating rates illustrated in Figure 3 shows that loss of crystalline structure for acetylsalicylic acid meets none of the requirements for thermodynamic (True) melting and is therefore an apparent melting material. A more accurate term than “melts with decomposition” would be “loss of crystalline structure due to a kinetic process” or Apparent Melting. For new users of DSC, the assumption may be that the shift in peak onset temperature with heating rate for Acetylsalicylic Acid is an instrumental effect caused by thermal lag. This is easy to disprove with a known melting point standard such as indium or phenacetin, a purity standard obtained from NIST (Figure 4). The effect of the change in heating rate from 1 to 20 °C/min is about 0.3 °C as compared to 10 °C with Acetylsalicylic Acid.
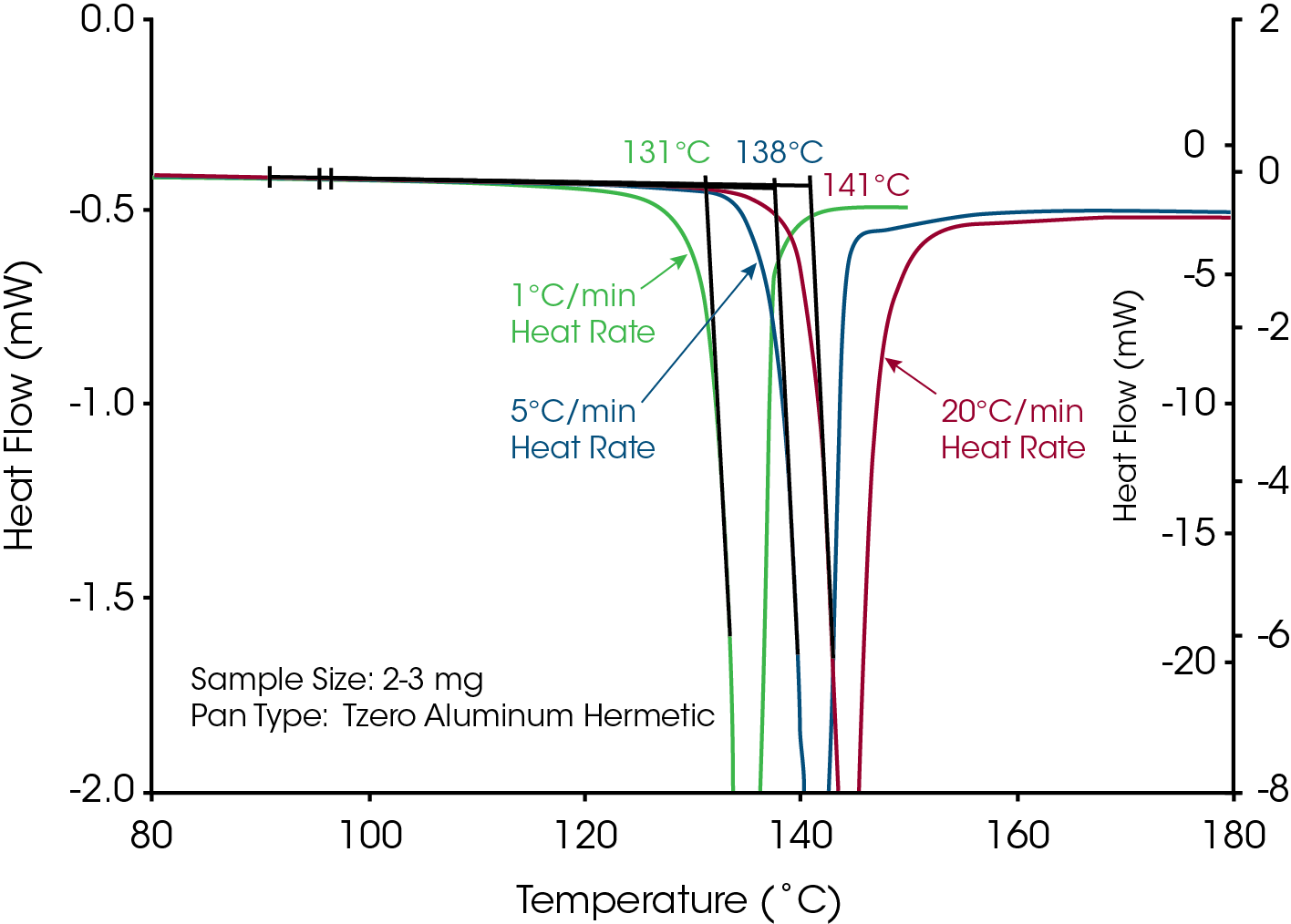
Before we consider other chemical processes that cause loss of crystalline structure, it is necessary to answer the question “If the endothermic peak is not True Melting then why does it look like a typical DSC melting peak?”. The answer to that question is not complicated and is based on the First Law of Thermodynamics, which states that energy cannot be created nor destroyed. As illustrated in the enthalpy plot of Figure 5, there is an absolute difference in enthalpy between crystalline and amorphous structure. If any process (thermodynamic, mechanical, or chemical) causes conversion of crystalline to amorphous structure then the material must absorb the absolute difference in enthalpy between the two phases at that temperature. The absorption of that energy difference would appear as an endothermic peak in the DSC curve, regardless of the cause.
Enthalpy plots are created by taking the absolute integral of heat flow rate (W/g) relative to time, or heat capacity (J/g°C) relative to temperature, over the temperature range of interest.
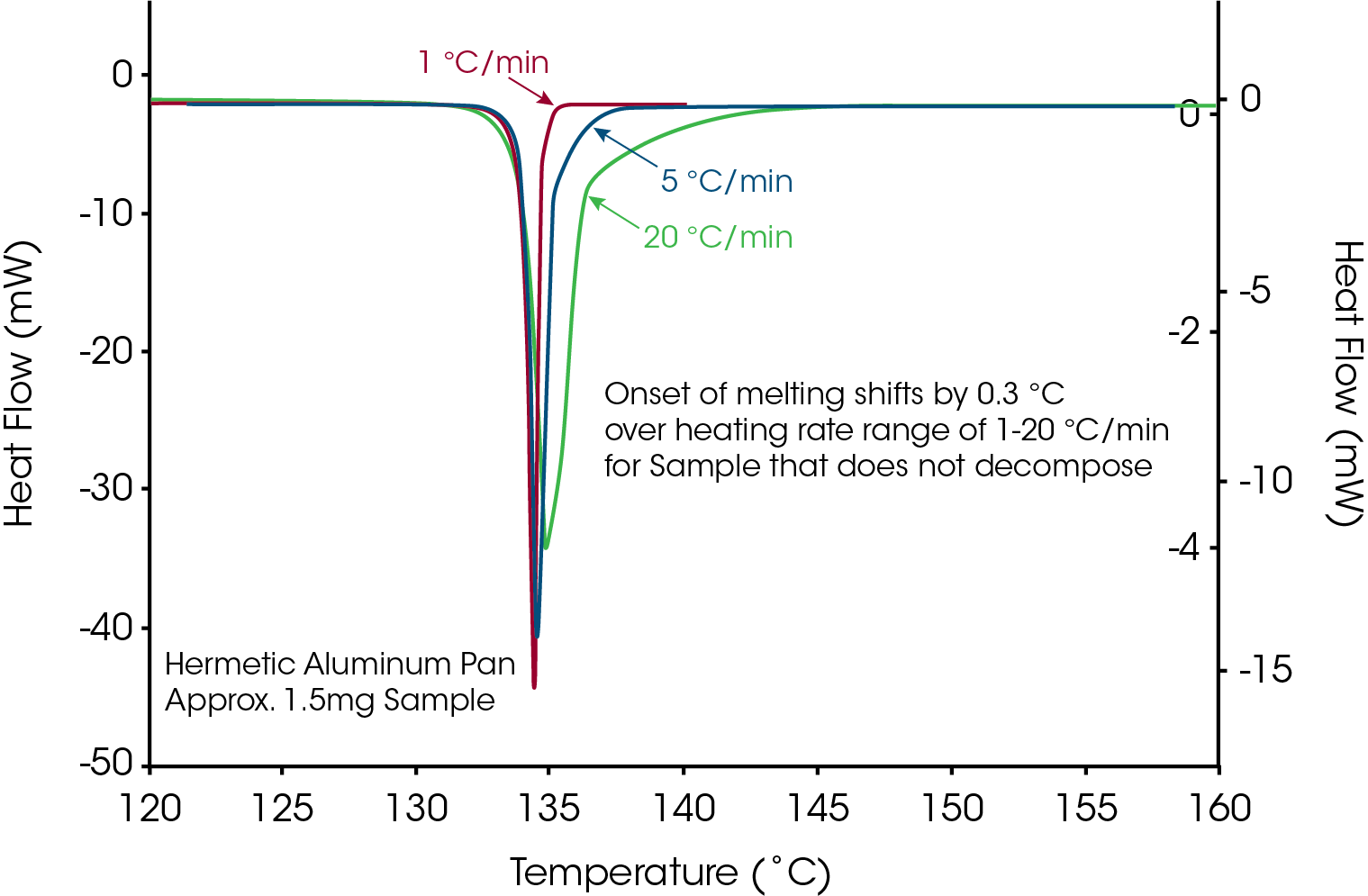
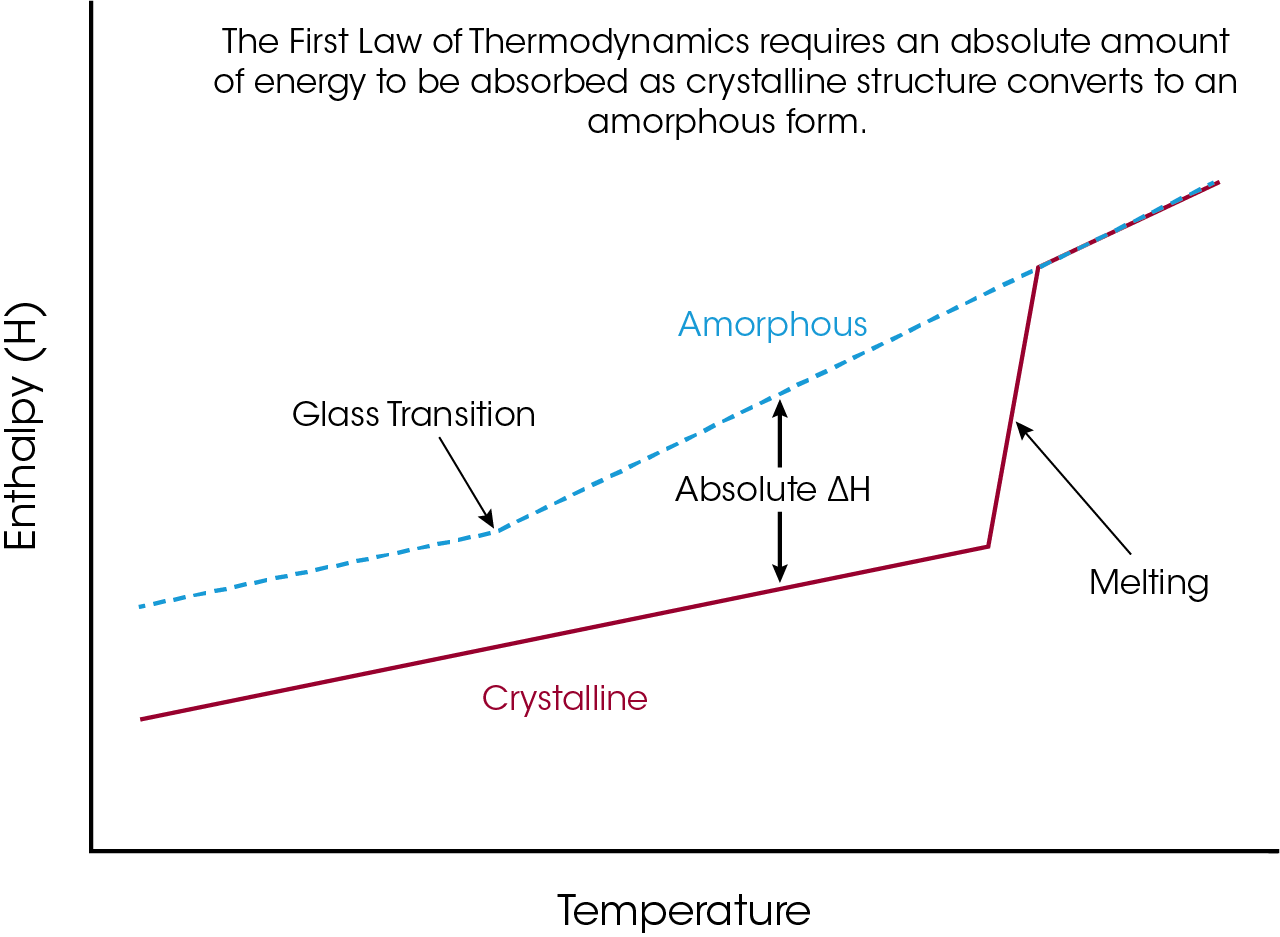
Other Causes of Apparent Melting
There are numerous chemical processes, other than decomposition, which can cause loss of crystalline structure and create an Apparent Melting peak in DSC data. They include:
Chemical Interaction
Pharmaceutical companies must always deal with the issue of drug-excipient incompatibility where the active pharmaceutical ingredient (API) reacts with a component of the formulation and results in a change in potency with time. The API is typically crystalline in order to improve stability and reduce chemical interaction. One of the most studied and reported interactions (Figure 6) is between magnesium stearate and acetylsalicylic acid (aspirin).
As these materials are easy to obtain and safe to use in the laboratory, anyone interested in Apparent Melting or drug-excipient interaction is encouraged to obtain these materials and do experiments similar to that seen in Figure 7. The DSC experiments include analysis of the individual components and then a 50/50 mixture. It is important to note that when the materials were mixed, no visible reaction was detected at room temperature. After mixing, a few milligrams were loaded into a hermetic DSC pan and then heated at 1°C/min, which was the same rate used for the individual components. The slow heating rate of 1°C/min was used to provide adequate time for the interaction to occur as temperature was increased. The small peak near 90°C in magnesium stearate was due to a small amount of hydrated form which was identified on the label of the container.

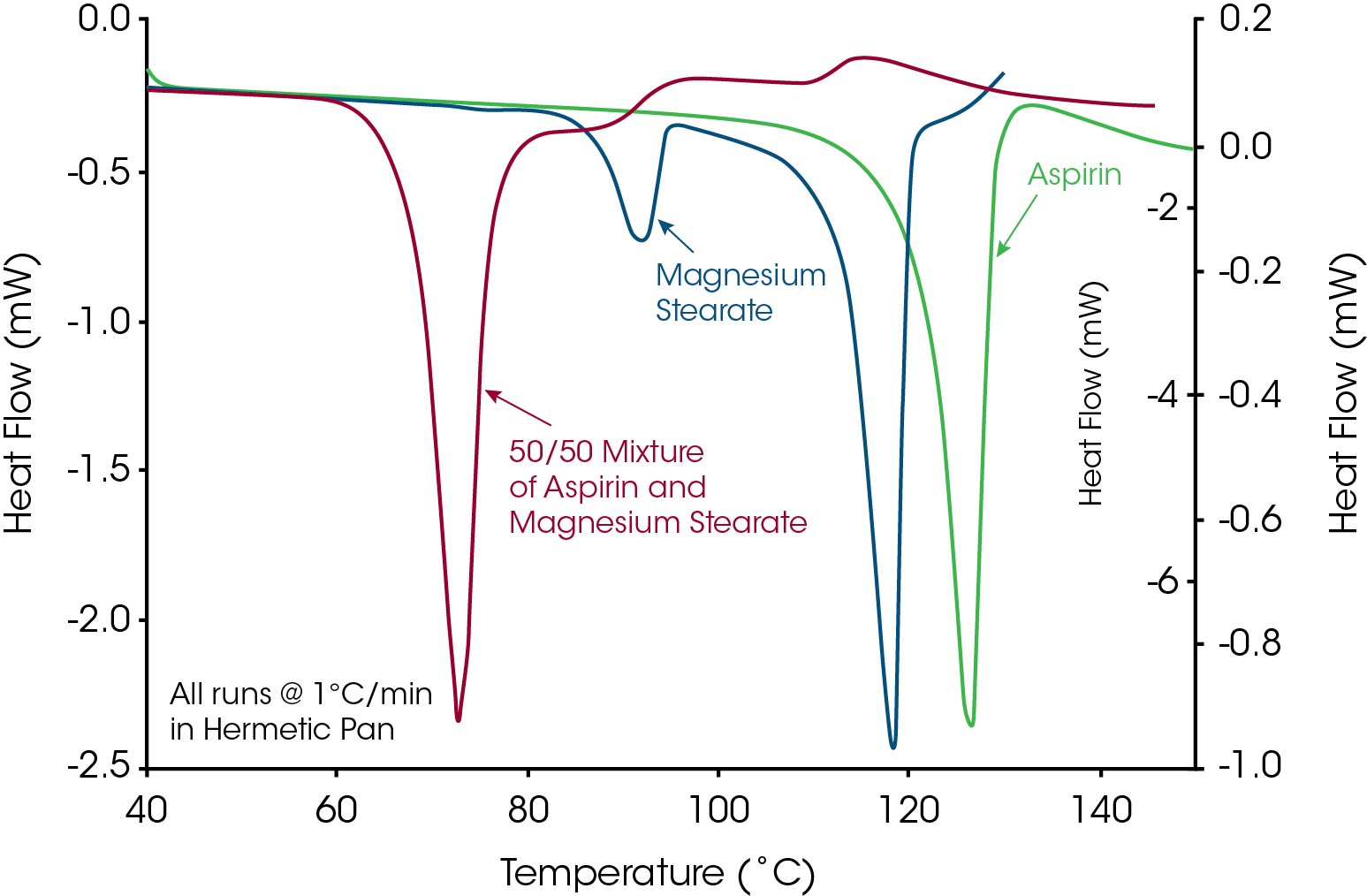
Desolvation
Dehydration, the most common form of desolvation, is the removal of a water molecule from a crystalline hydrate with the breaking of a covalent bond. The breaking of the bond disrupts the crystalline lattice and typically results in an amorphous anhydrous form and evolved water. Figure 8 compares the effect of pan type (hermetic vs. non-hermetic) on the dehydration of a crystalline hydrate with 5% bound water. The data from the non-hermetic pan (hermetic pan with pinhole) shows a broad endothermic peak due to evaporation of the water lost during the dehydration process. This is followed by a sharp exothermic crystallization peak near 120 °C which is crystallization of the amorphous material to form one of several possible polymorphic forms that then melts near 170 °C. Loss of water cannot occur in the hermetic pan and this prevents dehydration long enough to reach 100 °C, where crystalline structure is lost.

Dissociation
Dissociation is a general process seen in ionic compounds, such as salts, where the crystalline molecule is broken into smaller particles. As expected, this disrupts the crystalline lattice and causes a loss of crystallinity. An example of dissociation is seen in Figure 9 for Ciprofloxacin Hydrochloride. Even though the dissociation process is very exothermic, it is very important to notice that the first observed event is an endothermic peak. As required by the first law of thermodynamics, a material must absorb the enthalpy difference between the crystalline and amorphous states as it begins to lose crystalline structure.

Thermal Decomposition
Thermal decomposition was discussed and illustrated in the Introduction section for acetylsalicylic acid, but a significant amount of research has been done and published4 on the loss of crystalline structure in sugars, especially sucrose. The shift in peak onset temperatures in Figure 10 is due to hydrolysis (kinetic process) that starts within mother liquor occlusions in the cane sucrose crystal.
Figure 11 illustrates a very interesting approach to understanding chemical changes that occur during loss of crystalline structure in the sucrose. As stated in the Wunderlich definition, thermodynamic melting occurs “without chemical change”. This approach uses Modulated DSC (MDSC®) to follow loss of crystalline structure as a function of time at 120 °C and HPLC to analyze for thermal decomposition products of samples.

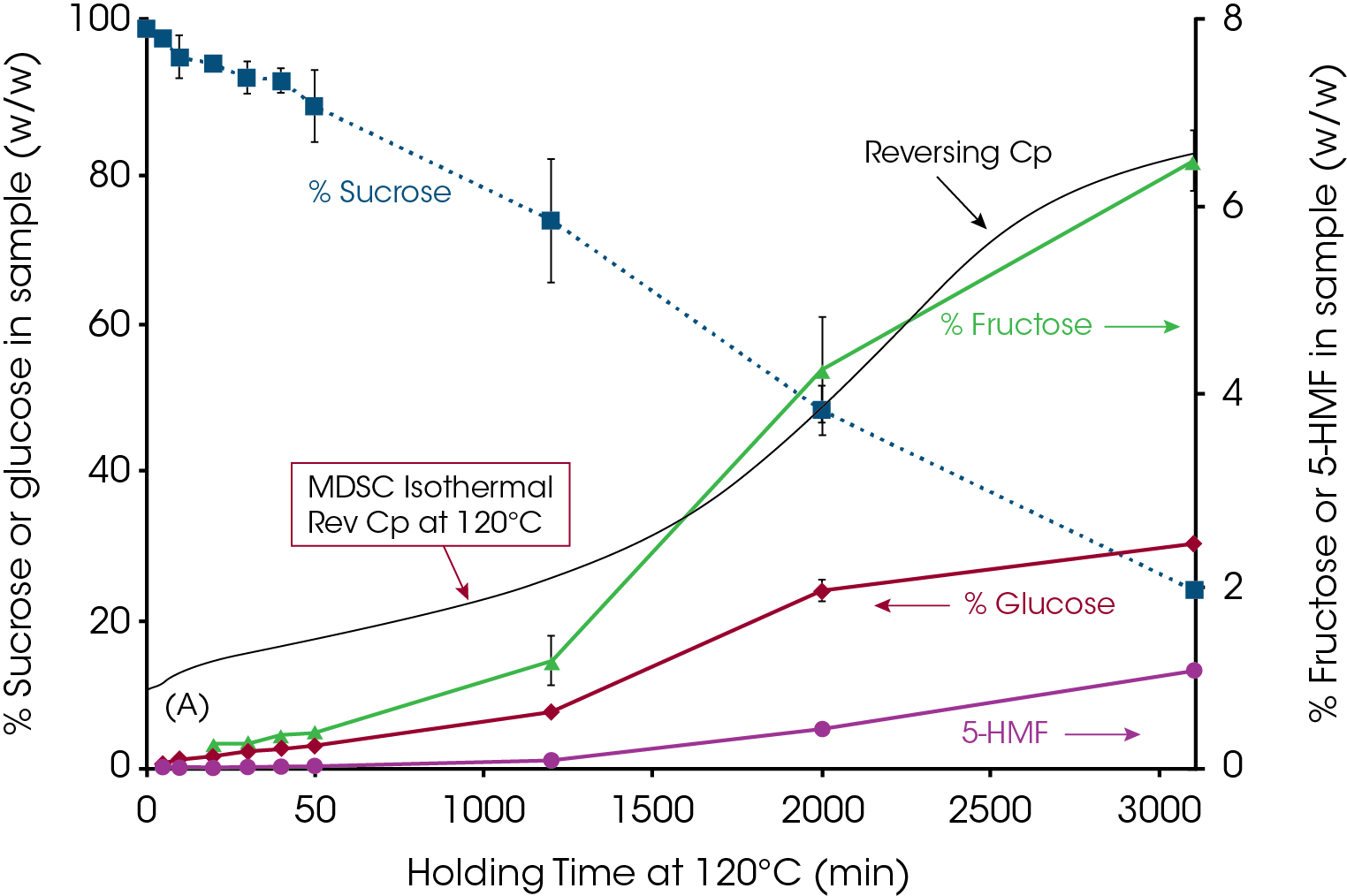
Importance of Distinguishing Between True and Apparent Melting
Some may think that it is not important to distinguish between True and Apparent Melting since crystalline structure is converted to amorphous structure in both cases. This is not a good assumption for the reasons below. These are the limitations imposed on the data from loss of crystallinity due to Apparent Melting, which is always the result of an underlying kinetic process.
1. Cannot Measure Accurate (Thermodynamic) Melting Point
Endothermic peaks due to Apparent Melting change with heating rate due to a kinetic process. The amount of change varies from material to material and is a function of the activation energy of the process. This can be seen in Figure 12 from a paper of Magon et al.5 where melting temperature is plotted against heating rate for both the small and large peaks seen in sucrose. The conclusion reached by Magon et al. in the paper is that the equilibrium melting point is 424.4°K (approx. 151°C) as compared to reported values as high as 190°C. This clearly demonstrates the problem of measuring the melting point of materials that undergo Apparent Melting.
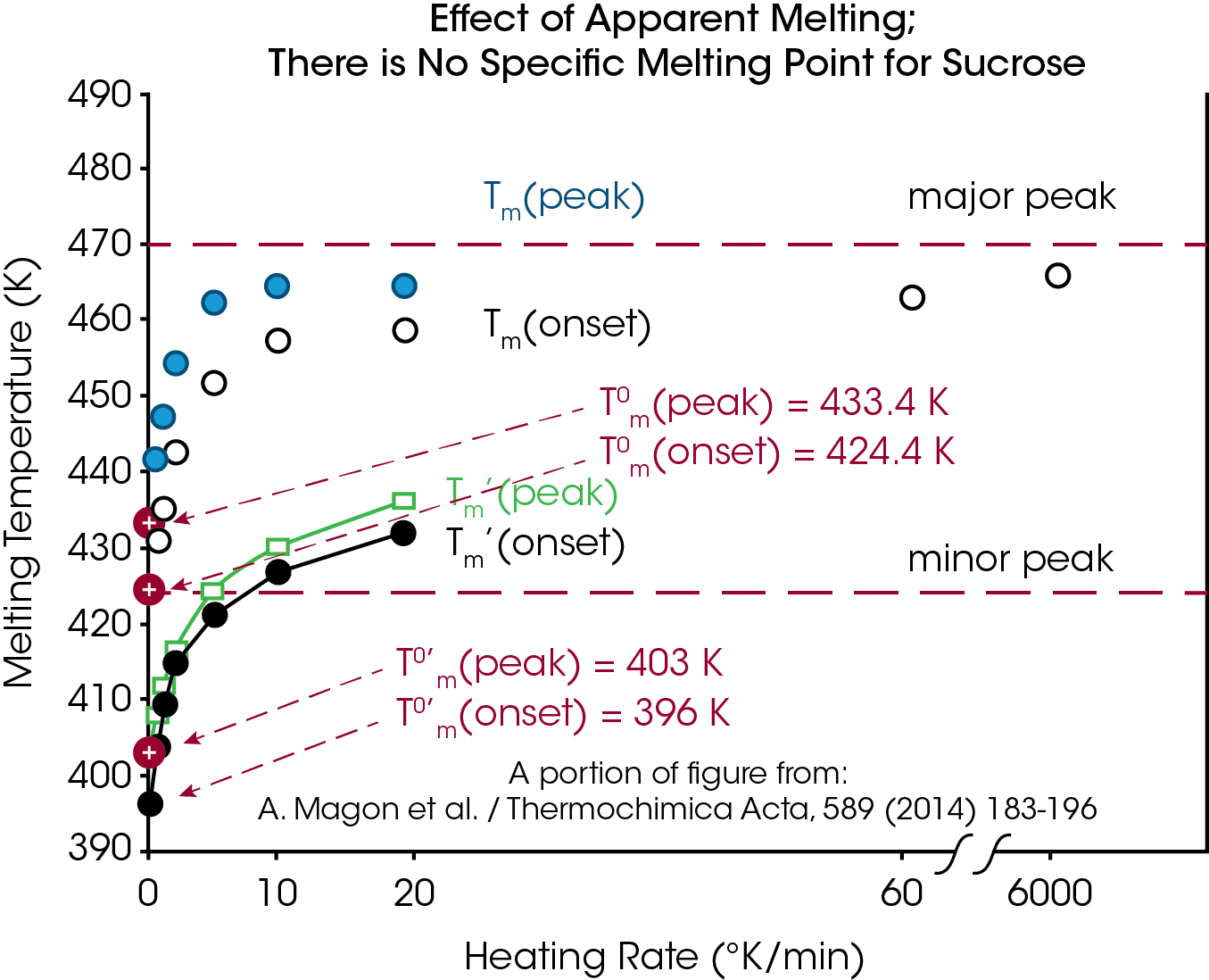
2. Cannot Measure Accurate Heat of Fusion
Just as the melting point cannot be accurately measured with materials that undergo Apparent Melting, it is not possible to measure an accurate heat of fusion. In the event of Apparent Melting the heat contribution from the underlying kinetic process renders the measured enthalpy a temperature dependent variable which is not the case for a true melt transition. This is illustrated in Figure 13 from Magon et al.
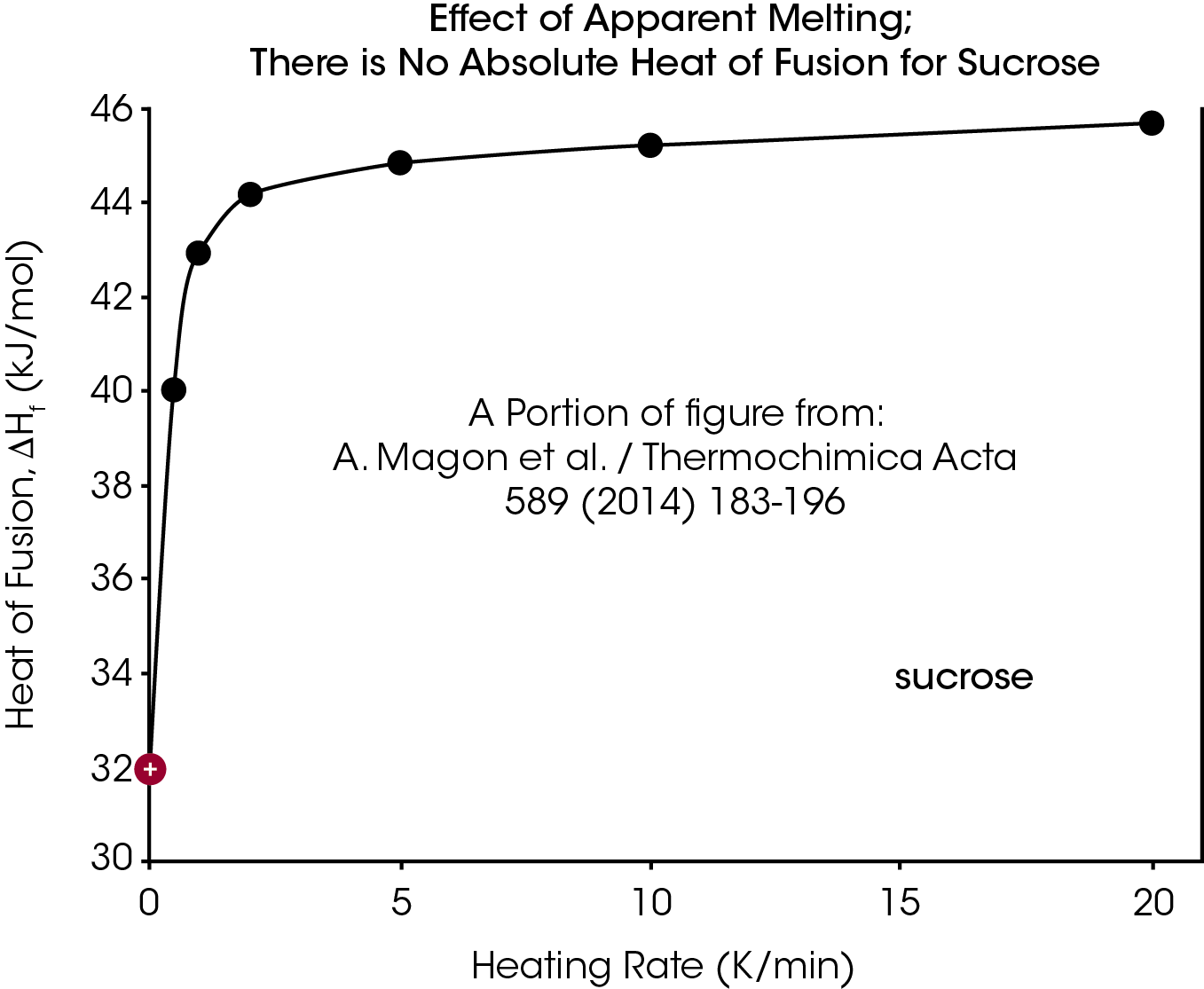
3. DSC Purity Cannot Be Measured
DSC purity analysis requires thermodynamic melting in order to detect and quantify impurities. In addition, the purity measurement is typically done at a low heating rate, such as 1°C/min, to ensure thermal equilibrium throughout the sample. Apparent melting creates impurities because of the thermal decomposition process that is causing the loss in crystalline structure.
4. Accurate Glass Transition Temperatures Cannot be Obtained from Melt-quenched Materials that Undergo Apparent Melting
If loss of crystalline structure is caused by a chemical process, as happens with Apparent Melting, the chemistry of the cooled sample is different than the original crystalline material. The change in chemistry creates smaller molecules and typically results in a lower glass transition temperature than expected for an amorphous material that has not been partially decomposed.
5. Identification of Polymorphic Forms Can Not Be Based on Melting Point
Polymorphs have the same chemical structure, but different crystalline structure and different melting points, which may differ by only a few degrees. Materials that undergo Apparent Melting do not lose crystallinity at specific temperatures and therefore the data cannot be used to identify polymorphic forms.
Conclusions
As illustrated with a range of materials, it is important to distinguish between True (thermodynamic) and Apparent (kinetic) Melting in order to obtain accurate and reliable results that can be correctly interpreted. It is very easy to make the determination by simply doing experiments at two heating rates (2 and 10 °C/min are recommended) on crystalline samples that have not been previously fully characterized. If the difference in onset temperature between the two rates is less than 1 to 2 °C then the material is showing thermodynamic melting behavior. If the difference is larger, then rates of 1 and 25 °C/min should be done to prove the presence of a kinetic process that is causing Apparent Melting.
The Wunderlich definition is very helpful because it contains a set of criteria that can be used for interpretation of DSC data. It is suggested that the terms True and Apparent Melting be used when reporting results either internally or in publications.
References
- Lee, J.W. et al.; Investigation of the heating rate dependency associated with the loss of crystalline structure in sucrose, glucose, and fructose using a thermal analysis approach (Part I). J. Agric. Food. Chem. 2010. DOI: 10.1021/jf1042344
- Lee, J.W. et al; Investigation of Thermal Decomposition as the Kinetic Process That Causes the Loss of Crystalline Structure in Sucrose Using a Chemical Analysis Approach (Part II). J. Agric. Food. Chem. 2010. DOI: 10.1021/jf1042344
- Lu, Y. et al. Impact of Sucrose Crystal Composition and Chemistry on its Thermal Behavior, Journal of Food Engineering 214: 193-208 (2017)
- Wunderlich, B. Thermometry. In Thermal Analysis, 1st ed.; Academic Press: San Diego, CA, 1990; Chapter 3, pp 79-121.
- A Magon et al.; Heat Capacity and transition behavior of sucrose by standard, fast scanning and temperature-modulated calorimetry, J. Agric. Food Chem. 2011, 59, 702–712 DOI:10.1021/jf104235d
Acknowledgement
This note was written by Leonard C. Thomas, DSC Solutions and Shelly J. Schmidt, Ph.D., Professor of Food Chemistry, University of Illinois at Urbana-Champaign
Special thanks to the following researchers at the University of Illinois Urbana-Champaign, Department of FSHN for use of their work in this presentation:
- Joo Won Lee, PhD.
- Yingshuang Lu, PhD.
Click here to download the printable version of this application note.