Keywords: dynamic mechanical analysis (DMA), modulus, humidity, sustainable polymers, glass transition
TA461
Abstract
Bio-derived and biodegradable polymers are increasingly important materials that serve to improve the sustainability of a product from initial synthesis and manufacturing to end of life. However, in the presence of humidity the properties of these materials can change greatly, from the softening point to the mechanical characteristics. With humidity chambers, DMA and rheology can give insights into the changes that occur with moisture, thus greatly expanding the characterization and understanding of these materials. The TA Instruments DMA 850 equipped with a relative humidity unit provides the ability to comprehensively study the various effects of moisture on bio-derived polymers while they are in the solid state. In this apps note, the properties of PLA are studied under various controlled humidity and temperature conditions.
Introduction
Bio-derived and biodegradable polymers play an increasingly important role in mitigating industrial pollution associated with petroleum-based and/or non-recyclable polymers. Poly-lactic acid (PLA), which is a semicrystalline polyester that can be both bioderived and biodegradable depending on the synthesis mechanism, has grown in popularity in recent years.[1,2] It is one of few bioderived polymers that feature tensile properties comparable to traditional thermoplastics. Additionally, PLA is suitable for applications requiring biocompatibility such as implants, meshes, tissue scaffolds in addition to applications such as packaging, and consumer products. [1]
An important consideration of bio-derived and biodegradable polymers such as PLA is analyzing the overall affinity for water and the effects this may have on performance, such as mechanical properties and changes in sample dimensions.[3] Atmospheric moisture (relative humidity) can have the effect of plasticizing the polymeric material, effectively decreasing the elasticity, altering the mechanical performance of the polymer as a function of deformation frequency, and causing swelling (dimensional changes).[3] Unfortunately, these effects cannot be captured in a typical DMA or rheology experiment lacking humidity control, where typically only the temperature or atmospheric gas can be modulated. However, with the relative humidity fixture for the DMA 850, the complex mechanical properties of solid and/or rubbery materials can be characterized at a variety of environmental conditions, with the ability to modulate both temperature and humidity.
In this applications note, dynamic mechanical analysis (DMA) and rheology are used to characterize PLA’s response to humid environments, including the dependence of the glass transition temperature on humidity, mechanical properties as a function of frequency at various temperatures and humidity setpoints, and the expansion or contraction of a sample in response to moisture.
Experimental
The grade of PLA used in this work was LX175 purchased from Filabot (Vermont, USA). DMA samples were rectangular compression molded films, 7.5 mm long, 5 mm in width, and 0.35 mm in thickness. The DMA film tension clamp was used in all experiments in conjunction with the RH chamber add on. The operating range of the RH chamber can be found in Figure 1. To measure the effect of moisture on glass transition temperature, the samples were equilibrated at 25 °C, then conditioned at a humidity of 5, 30, 55, or 80% RH% for 300 minutes, and finally subjected to an oscillation temperature ramp of 1 Hz at 0.05% strain from 25-85 °C at a rate of 1 °C/min. The viscoelastic response of the PLA at 5, 30, 55, and 80% RH was measured by again doing thermal and humidity conditioning steps, following by an oscillation frequency sweep of 0.1-10 Hz at 0.05% strain. Finally, hygroscopic expansion was investigated by performing an oscillation time sweep after thermal conditioning to a temperature of 25, 37.5, or 50 °C. Samples underwent 1 Hz at 0.05% strain for 300 minutes at a set humidity of either 5, 30, 55, or 80% RH. After the sweep completed, the humidity was conditioned to the next setpoint at a rate of 2% RH/min and another oscillation time sweep was performed.
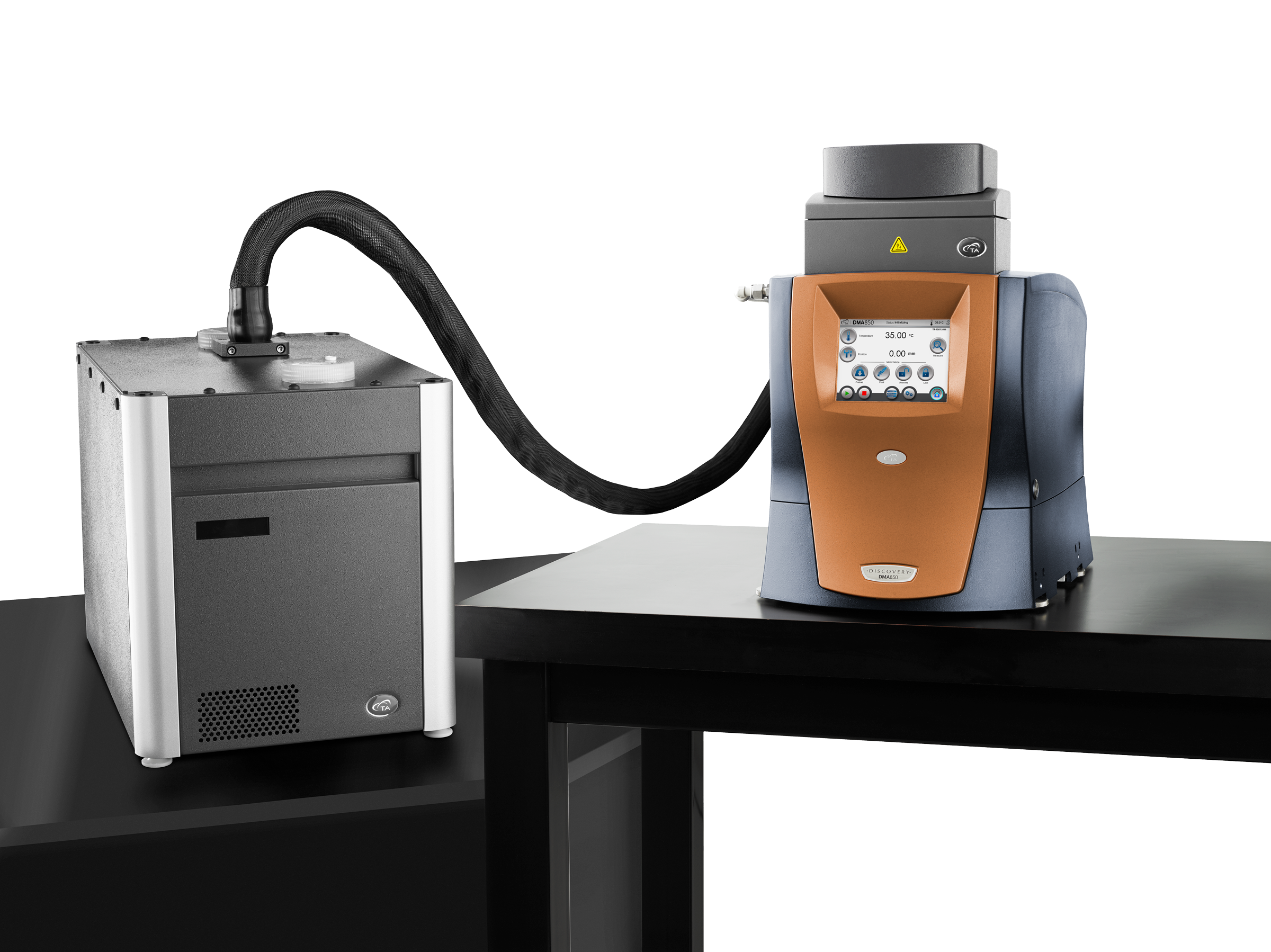
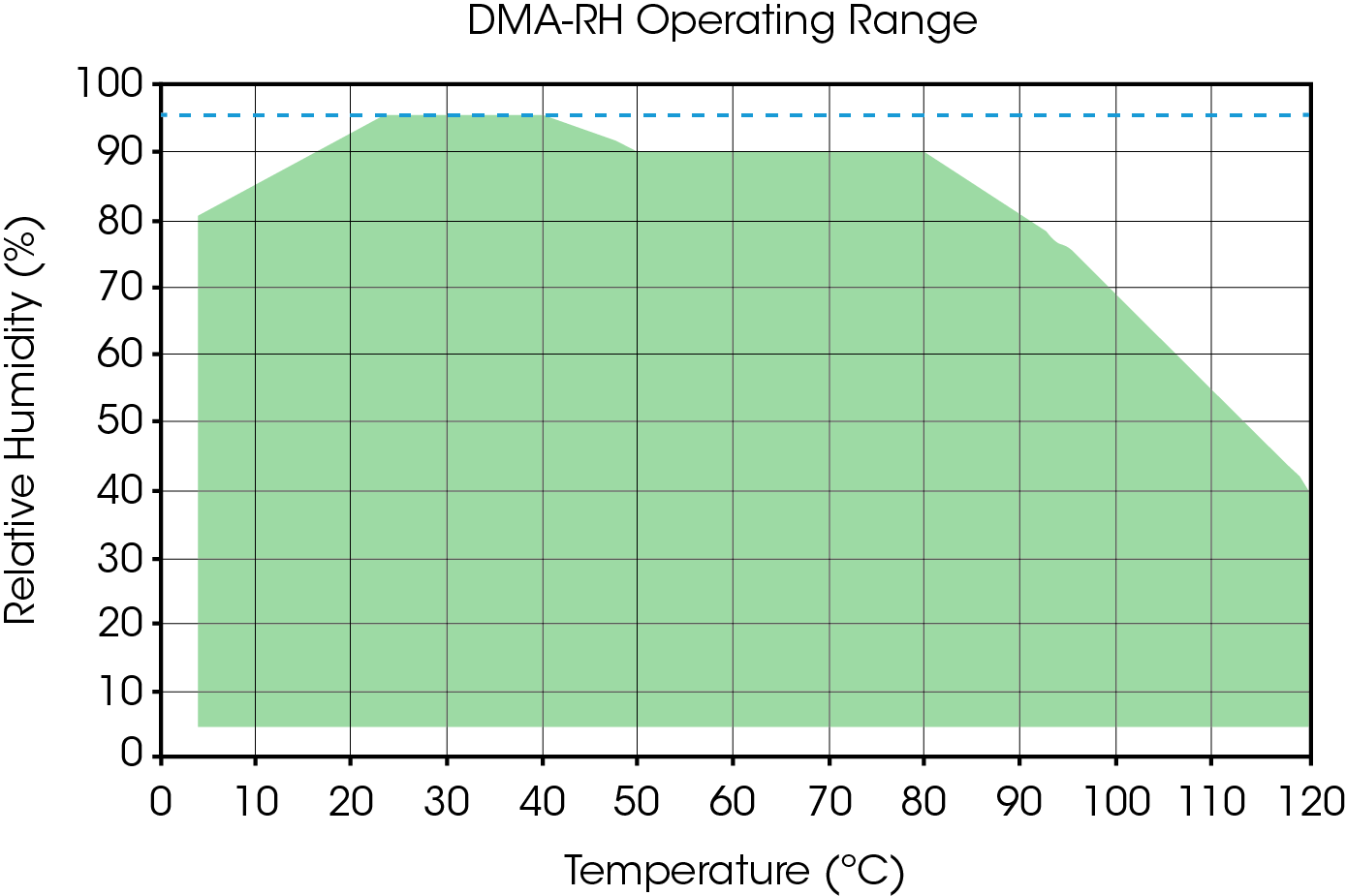
Results and Discussion
Glass Transition Dependence on Humidity
Figure 2 shows oscillation temperature ramp data, where tan delta is plotted as a function of temperature for various humidity setpoints. Moisture acts as a plasticizer to PLA, effectively lowering the strength of the molecular interactions of the polymer matrix, thus lowering the glass transition. [4,5] The peak points of the tan delta represent one method of determining the glass transition temperature. As humidity increases, the effective glass transition temperature decreases. Between a relative humidity of 5-80%, the glass transition temperature varies over 10 °C (62-73 °C). This is a major consideration for not only processing various grades of PLA, but also the softening point (upper thermal limit, heat deflection temperature) which has implications for the final product. This effect is well known for Nylon, among other polymers, but is also of critical importance for bio-derived and/or biodegradable polymers.[4] Polymer processers can use this information to observe how humidity may be introducing variability to their process, or to condition a polymer to a humidity level to take advantage of the plasticizing effect of the moisture.
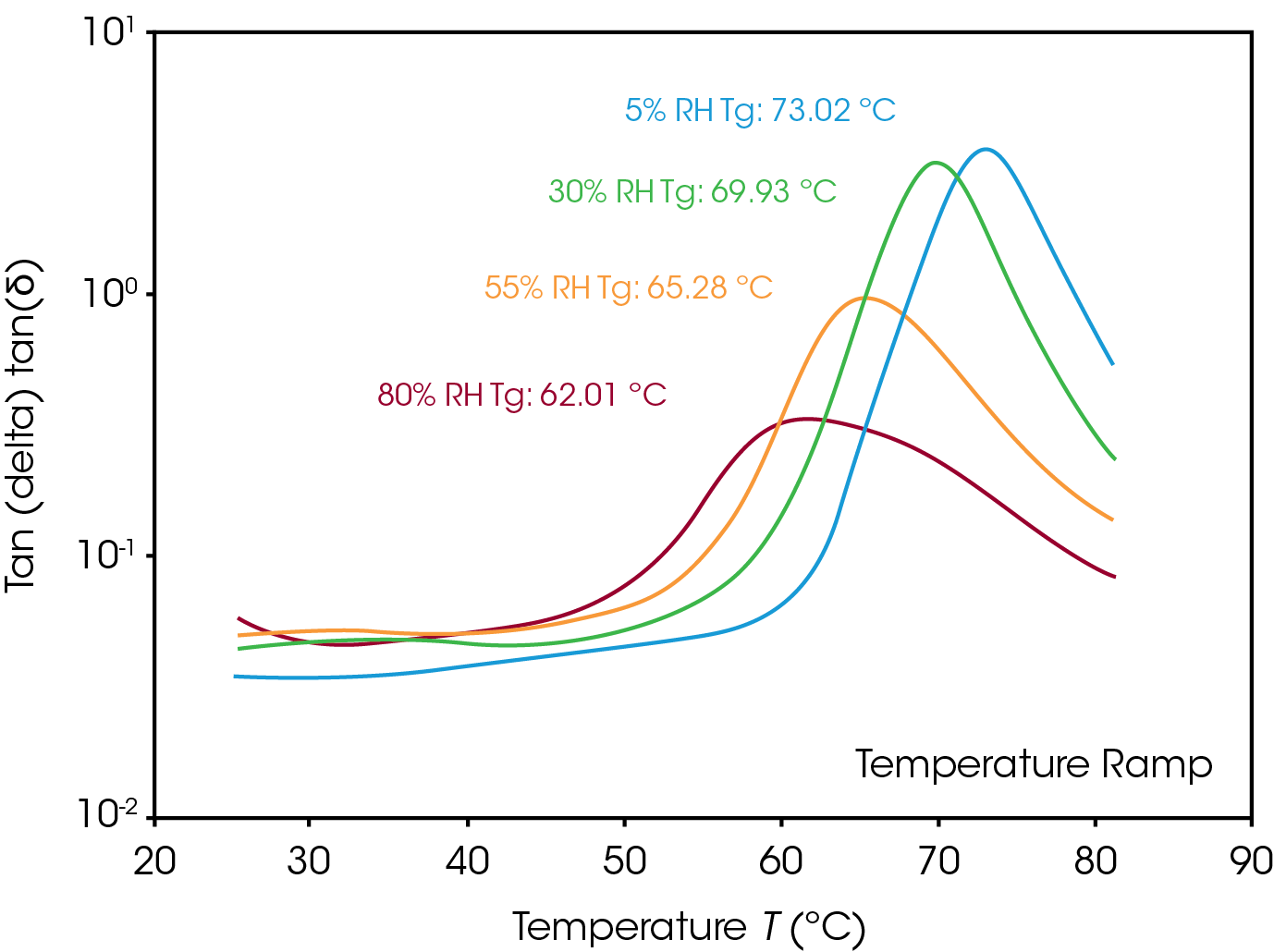
Viscoelastic Response to Moisture at Different Temperatures
Frequency sweeps provide complex viscoelastic data such as storage modulus, loss modulus, tan delta as a function of the deformation frequency, effectively measuring the mechanical properties, or the extent to which a material responds in a liquid or elastic modality and related molecular relaxation processes as a function of timescale or frequency. The viscoelastic data as a function of frequency for the PLA films has been characterized at a variety of humidity settings (5, 30, 55, 80% RH), and for three overall temperatures all below the glass transition of the PLA (25, 37.5, and 50 °C relative to a glass transition temperature range of 62-73 °C).
Figure 3 features an overlay of frequency sweep data at each humidity setpoint, at a testing temperature of 25 °C. Storage modulus and tan delta are both plotted as a function of frequency. As a result of increased humidity, storage modulus decreases slightly and tan delta increases, which both demonstrate the softening of the PLA. It is worth noting, however, that the changes are very small in magnitude at this temperature.
This contrasts with the results at 37.5 °C, shown in Figure 4, where again storage modulus and tan delta are plotted as a function of frequency for the various humidity setpoints. While changes to the storage modulus are still marginal with increased humidity, the changes to tan delta are much more substantial at this temperature. The increase in the tan delta with humidity indicates that the PLA is becoming less elastic and more viscous in terms of mechanical response.
Finally, in Figure 5 storage modulus and tan delta are plotted as a function of frequency for the various humidity setpoints, when the PLA sample is held at a temperature of 50 °C. At this temperature, the effect of humidity on the mechanical response is considerably larger than at the previous temperatures. At a frequency of 0.1 Hz, the change in storage modulus and tan delta are over a decade in magnitude, when considering humidity setpoints of 5% and 80%. Additionally, the complex mechanical response also has a much stronger frequency dependence, which wasn’t observed at the other temperatures.
This data illustrates that even below the glass transition temperature, the viscoelastic properties of semicrystalline PLA polymer still show sensitive response to the environmental temperature and humidity, where the polymer exhibits different mechanical properties than expected. These measurements allow a producer or manufacturer to define limits for the use of the material, which are dependent on the environment.
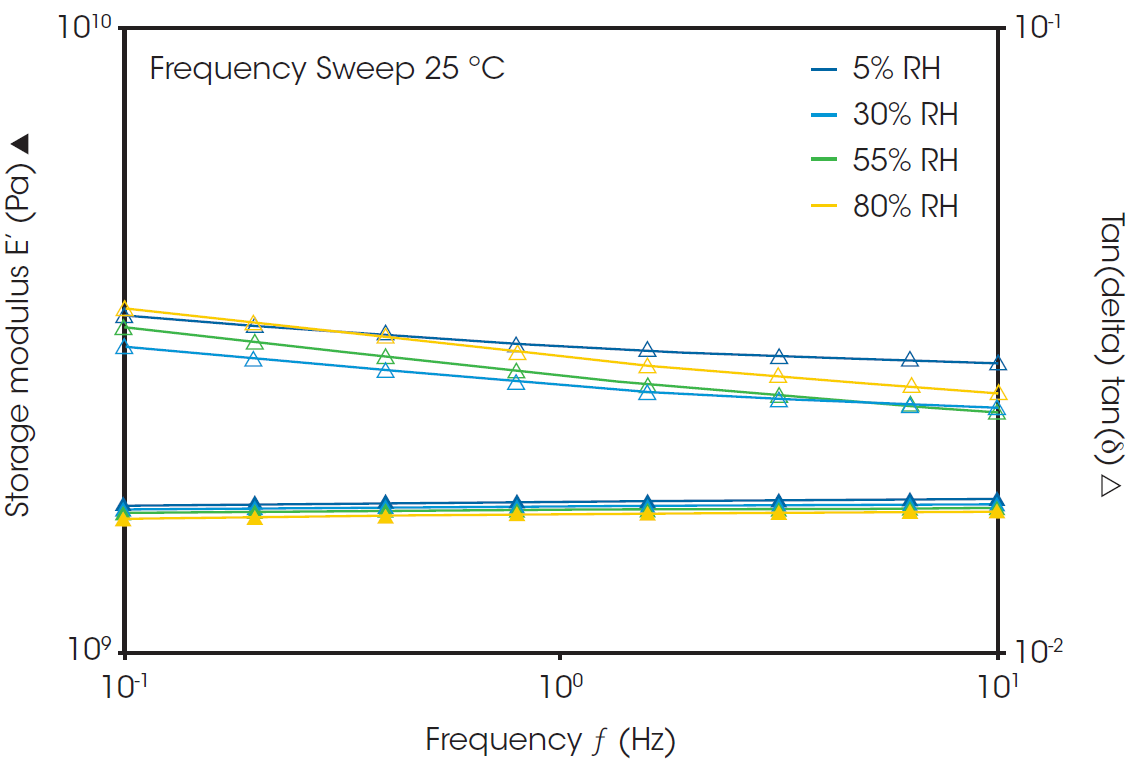
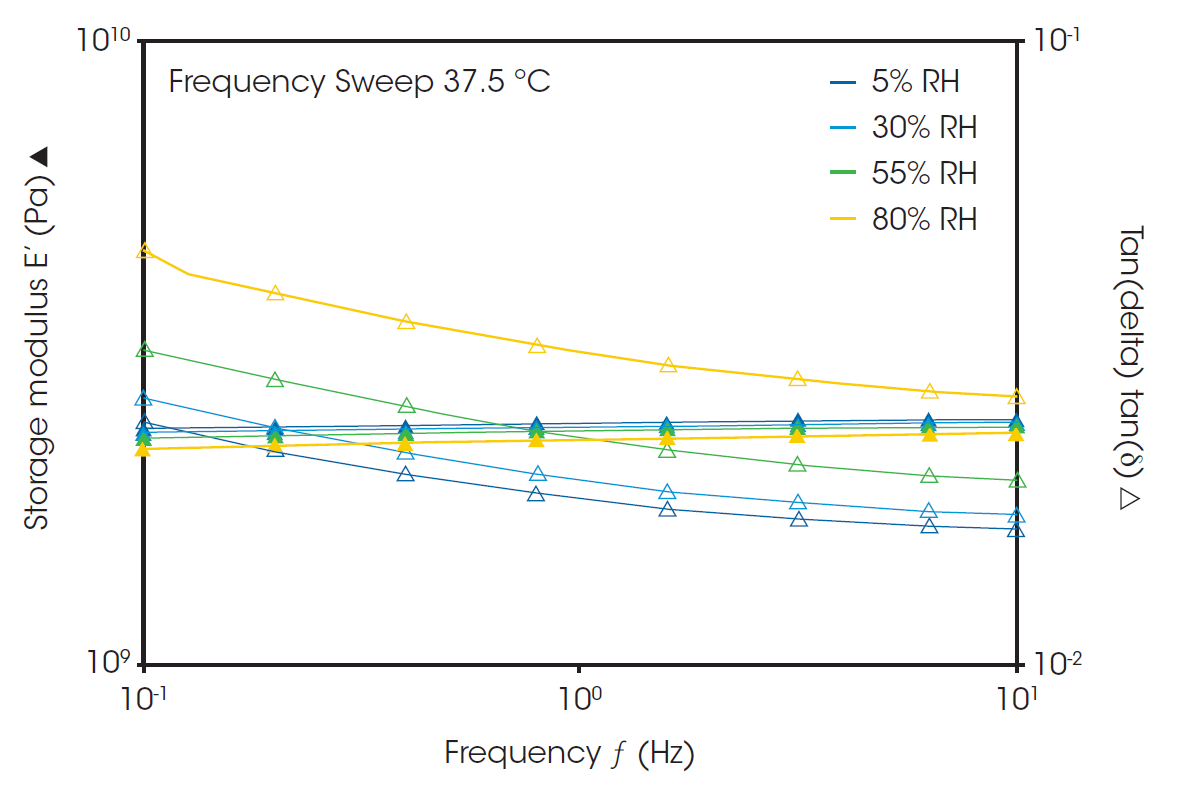
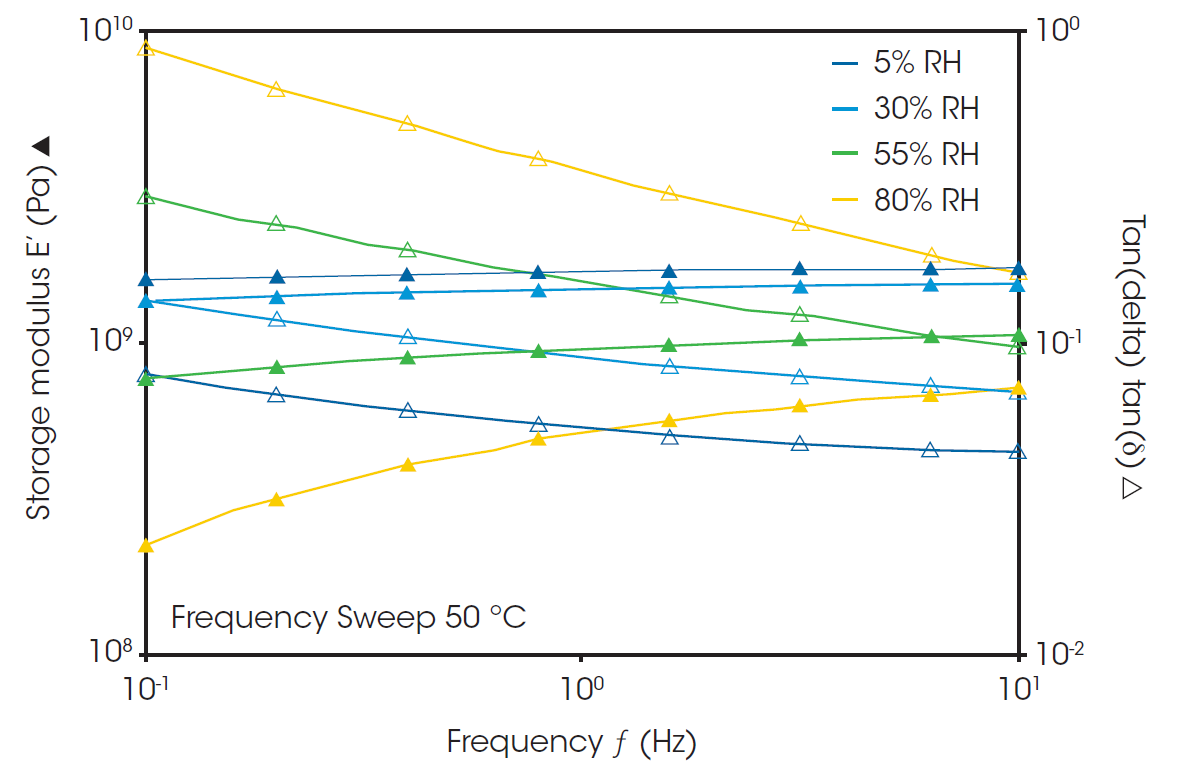
Hygroscopic Expansion
Analogous to the changes in mechanical response due to a combination of temperature and moisture as seen in the last section, the expansion or contraction of a material can also be characterized in response to these factors. From an oscillation time sweep step in procedure, the length change of the sample can be observed as a function of time.
Figure 6 features oscillation time sweep data, where sample length is plotted as a function of time for temperatures of 25, 37.5, and 50 °C respectively. The curves in each plot are representative of sample length after held at the setpoint environmental temperature and the various humidity setpoints. From the starting point of each curve, the humidity is ramped in increments every 300 minutes (see inset), the ramping period can be observed through small gaps in the curves.
At 25 °C, it can be observed that regardless of the humidity setpoint, the magnitude of sample expansion over the course of the observation window is negligible. When the temperature is increased to 37.5 °C, the sample undergoes a bit more elongation but the overall percent change is still quite small, expanding approximately 1% in length. This demonstrates that at these temperatures and humidity setpoints, the changes in the sample dimensions in still very small.
When held at 50 °C, the sample undergoes significant expansion at elevated humidity setpoints. At a humidity setpoint of 55%, around 800 minutes into the experiment, the sample elongates over 20% from the start to the end of the oscillation time step. This effect is accelerated at higher humidity setpoints, until the sample is saturated with moisture and the length starts to reach a plateau. Between 37.5 °C and 50 °C and above 30% relative humidity, the sample has major dimensional expansion compared to the lower setpoints. This sets an upper limit on the environmental conditions that this grade of PLA can be used in before the product geometry expands.
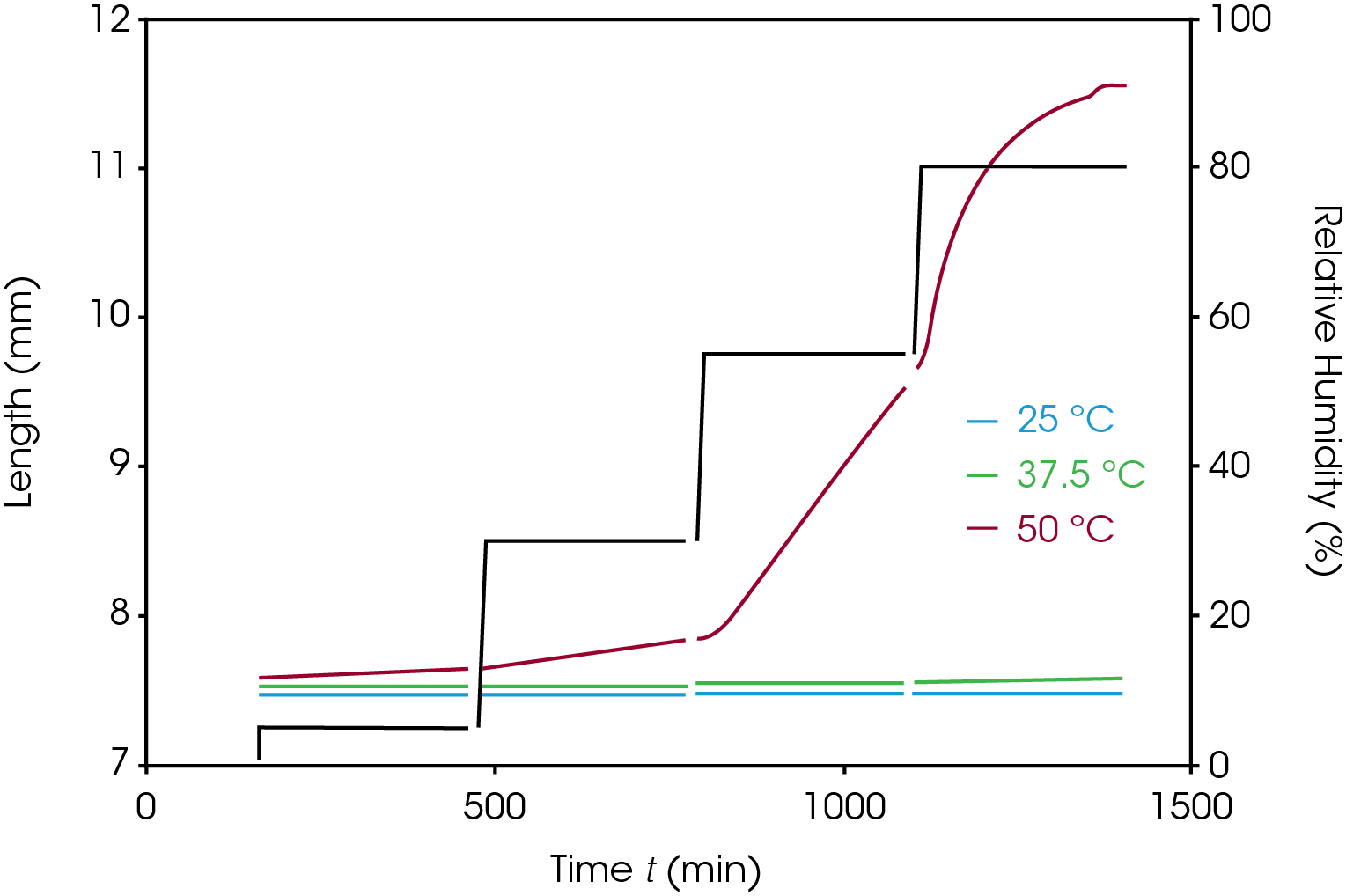
Conclusions
The TA Instruments DMA 850 equipped with the relative humidity chamber is a powerful tool and workstation to comprehensively characterize bio-derived sustainable polymers, as well as a host of other materials. In this applications note, the effect of moisture on the properties of a bioderived polymer (PLA) were characterized using three methods:
- One method characterized the glass transition temperature at different relative humidity setpoints. This can be of critical importance for polymer processing or in applications where the heat deflection temperature or upper thermal limit are critical.
- The next method characterized the frequency dependent mechanical properties at several temperatures and humidity setpoints. Depending on the timescale (frequency) of mechanical deformations applied to the polymer, the humidity, and the temperature, the polymer may have mechanical properties that differ from the material data sheet.
- The last method characterized changes in the dimensions of the PLA sample (percent length change) when subjected to a several temperatures and humidity setpoints. This is critical for final products, which are generally molded or machined to specific dimensions and tolerances
The data obtained from these methods offers powerful insight into the effect of moisture and humidity on the processing and performance of bio-derived polymers, a key understanding needed to produce and utilize these sustainable polymers.
References
1. Masutani, K., and Kimura, Y. “Poly(lactic acid) Science and Technology: Processing, Properties, Additives and Applications” The Royal Society of Chemistry, 2015, pp. 1-36
2. Tokiwa, Y., Calabia, B.P. Biodegradability and biodegradation of poly(lactide). Appl Microbiol Biotechnol, 2006, Vol. 72
3. Holm, V.K., Ndoni, S. and Risbo, J. (2006), The Stability of Poly(lactic acid) Packaging Films as Influenced by Humidity and Temperature. Journal of Food Science, 71: E40-E44. https://doi.org/10.1111/j.1365-2621.2006.tb08895.x
4. Rocca-Smith, J. R. and Whyte, O. and Brachais, C-H and Champion, D. and Piasente, F. and Marcuzzo, E. and Sensidoni, A. and Debeaufort, F. and Karbowiak, T. “Beyond Biodegradability of Poly(lactic acid): Physical and Chemical Stability in Humid Environments”. ACS Sustainable Chemistry & Engineering, 2017, Vol. 5
5. Grunina, N. A. and Belopolskaya, T. V. and Tsereteli, G. I. “The glass transition process in humid biopolymers. {DSC} study”. Journal of Physics: Conference Series, 2006, Vol. 40
Acknowledgement
This paper was written by Keith Coasey, Ph.D., Rheology Applications Engineer at TA Instruments.
Click here to download the printable version of this application note.