Keywords: Differential Scanning Calorimetry, High Throughput Thermal Stability Screening, Short Term Thermal Stability
MC177
Abstract
Biotherapeutic thermal stability testing is crucial to ensure product quality and support regulatory approval of biologic drug products. The TA Instruments™ RS-DSC (Rapid Screening-Differential Scanning Calorimeter) provides a platform for simultaneous analysis of up to 24 samples. Further, high concentrations of protein drug candidates can be evaluated using low-volume disposable microfluidic chips. This application note provides data illustrating the utility of the TA Instruments RS-DSC by evaluating the limit of detection and demonstrating the range of concentrations amenable to differential scanning calorimetry (DSC) analysis.
Introduction
Short-term thermal stability testing is essential in evaluating a compound’s resistance to thermal stress, which is key to predicting shelf life and maintaining efficacy. Differential Scanning Calorimetry (DSC) is a technique employed to understand antibody structure; however, formulation concentration samples are difficult to characterize in traditional instruments. These challenges stem from the use of an irreplaceable sample cell, necessitating lengthy cleaning steps to prevent sample carryover between scans. The fixed sample cell also limits the upper concentration range, as highly concentrated protein is more likely to clog the cell upon heating. In the best case, a clogged cell requires extensive, harsh cleaning to regenerate. At worst, a cell can be irreversibly contaminated. Thus, samples require dilution from their formulation concentrations. Concentration can affect the thermal stability of proteins, so an accurate understanding of protein stability for potential drugs under storage conditions requires the testing of formulation strength samples.
The TA Instruments RS-DSC is an innovative leap forward in differential scanning microcalorimetric technology. Unlike other DSCs, the TA Instruments RS-DSC does not require samples to be diluted because it is uniquely designed to handle high-concentration biologic drug formulations in low-volume and disposable microfluidic chips. This technological integration eliminates the need for repetitive cleaning of the instrument measurement cell between runs, saving time, reducing the risk of contamination, and enabling more reliable readings. A sample can be prepared, sealed, and ready for analysis in less than one minute, requiring only minimal volumes for precise evaluation. The TA Instruments RS-DSC redefines the thermal stability testing landscape by simultaneously analyzing 24 samples – a new platform for highthroughput thermal stability testing for the biopharmaceutical industry. Providing comprehensive physicochemical insights through thermal analysis can expedite the development of drug products to bring them to market efficiently.
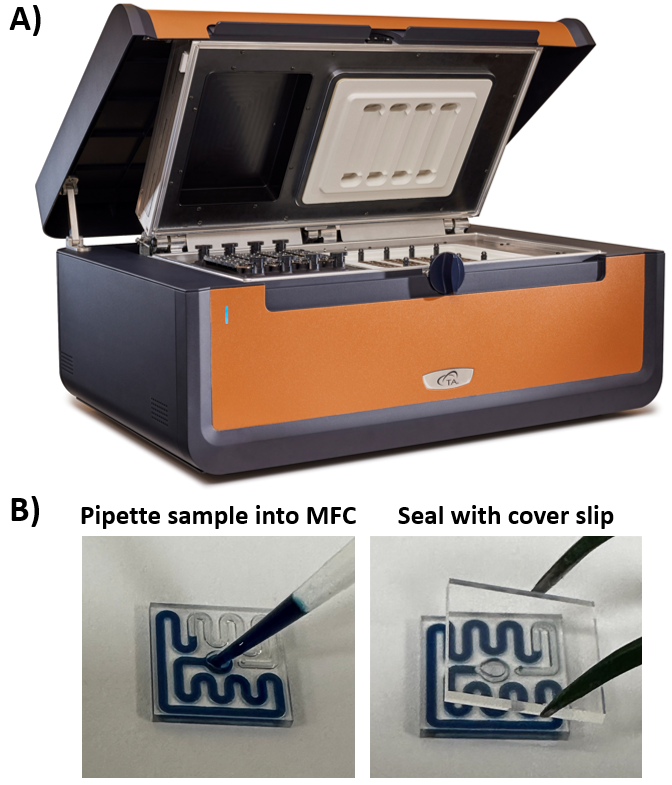
Experiments and Methods
Herceptin® Trastuzumab was prepared according to package instructions in histidine formulation buffer (18.4 mg/mL trehalose dihydrate, 0.08 mg/mL polysorbate 20, 0.49 mg/mL histidine HCl, 0.32 mg/mL histidine, pH 6.0) at 21 mg/mL and stored at 4 °C until use. For analysis in phosphate buffered saline (PBS), the antibody was buffer exchanged using an Amicon™ Ultra 10kDa molecular weight cut off centrifugal spin filter into PBS composed of Gibco™ DPBS with 1 mM EDTA at pH 7.4, adjusted to the desired concentration, and tested in triplicate.
Chicken egg white lysozyme used for evaluation of high concentration protein solutions was obtained from Sigma Aldrich (L6876) and formulated in glycine buffer (0.1 M glycine, pH 2.5). Protein samples were concentrated to 330 mg/mL using an Amicon Ultra 3kDa molecular weight cut off centrifugal spin filter. Dilutions were made from the concentrated solution. Experiments were performed on the TA Instruments RS-DSC in triplicate immediately after preparation of protein concentrations.
Protein sample is pipetted directly into the disposable glass microfluidic chips (MFCs) with a channel that is designed to contain 11 μL of liquid sample. After addition of protein solution, the MFCs are sealed with an adhesive-backed glass coverslip to contain the sample during heating to 100 °C and are ready to be placed into the TA Instruments RS-DSC (Figure 1). Assembled MFCs are placed onto the sample side of each twin calorimeter. A reusable polyetheretherketone (PEEK) chip remains on the reference side. Up to 24 samples may be run simultaneously with a maximum temperature range of 20 – 100 °C and pre-set scan rates of 1 or 2 °C/min. By eliminating the need for cleaning between scans, up to 96 samples can be run in a typical workday.
The TA Instruments RS-DSC, operated with TA Instruments RSDSCRun software, was set to equilibrate at the initial temperature for 1800 s prior to the start of each scan. Each sample was run in triplicate and scanned at 1 or 2 °C/min across the full temperature range (20 to 100 °C).
To ensure accuracy across all 24 calorimeters, the instrument was first calibrated and validated with dipalmitoylphosphatidylcholine (DPPC), with an accepted Tmax shift for each calorimeter of less than 0.2 °C from the expected literature value [1].
Data was processed using NanoAnalyze™ Software (v4.0.0). New software features allow for automated detection of the denaturation midpoint temperature (Tmax) and comparison of up to 96 thermograms. NanoAnalyze Software v4.0.0 includes a new software algorithm, RapidDSC, that will automatically detect Tmax in DSC thermograms by searching for peaks across the specified range of data. An automatic baseline is applied across the peak and used to further refine the Tmax temperature for improved accuracy. Automatic peak detection features display up to three peaks across the temperature range of interest. The automation features can be easily edited in the “Baseline and Tmax Editor” pop out window to narrow the window of detection, select a specific range for peak identification, or adjust the automated baseline and Tmax. Tabulated data from Tmax automation can be exported for easy comparison or is viewable in the Tmax visualization and overlay tabs in RapidDSC.
Results and Discussion
TA Instruments RS-DSC Limit of Detection
With low sample volume requirements, it is important to understand the concentration limits needed for sensitivity and accuracy of data. Faster scan rates result in increased signal intensity and can aid in analysis of protein concentrations at or near the limit of detection. To understand the concentration requirements for a multi-transition protein, the antibody Herceptin Trastuzumab was adjusted to varying concentrations in PBS and evaluated in triplicate at a scan rate of 2 °C/min (Figure 2 and Table 1).
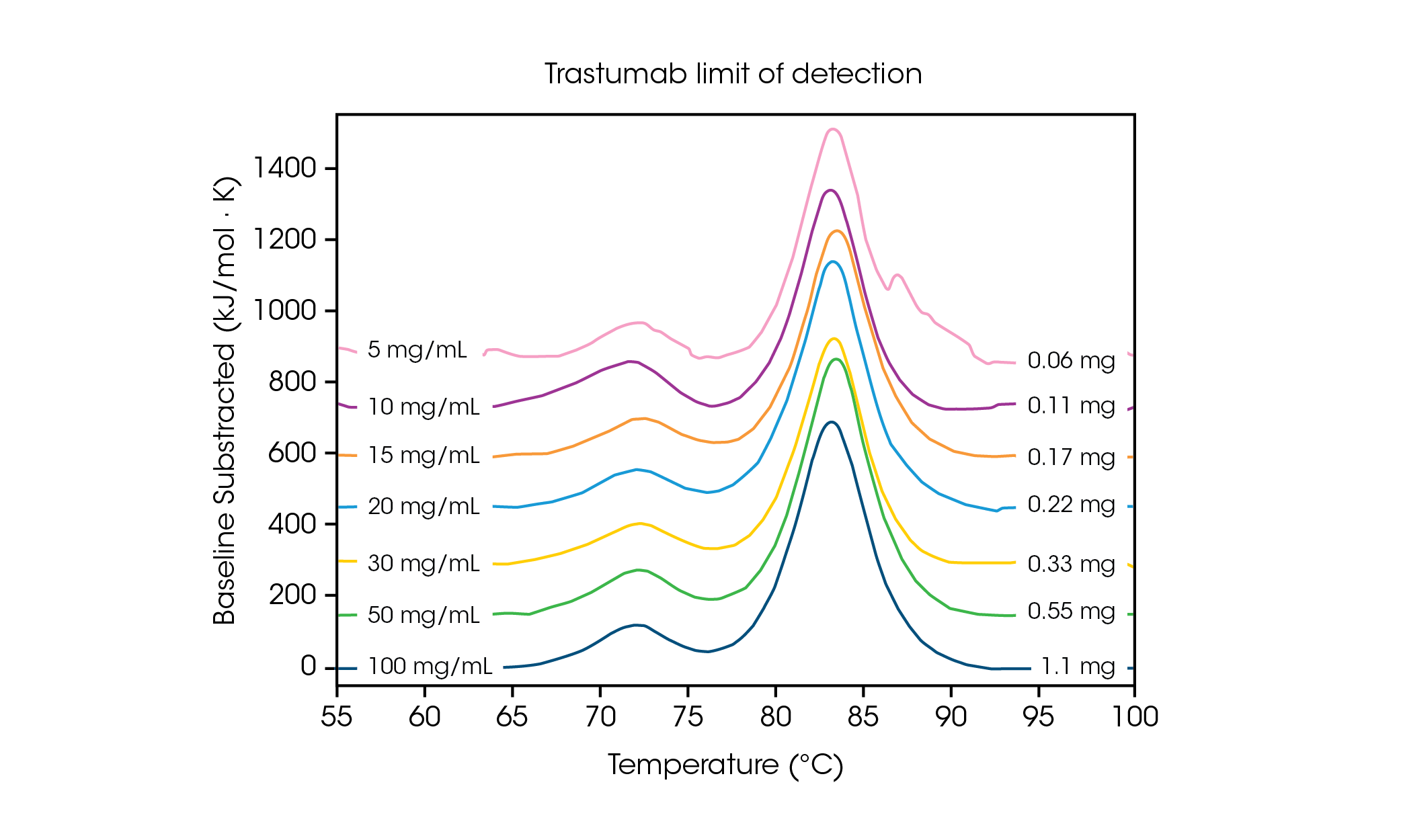
Table 1. Transition temperatures and reproducibility across limit of detection screen. Mean ± SD, n = 3.
Conc. | Tmax,1 (°C) | Tmax,2 (°C) |
---|---|---|
5 mg/mL | 72.57 ± 0.52 | 83.06 ± 0.09 |
10 mg/mL | 71.83 ± 0.29 | 82.98 ± 0.05 |
15 mg/mL | 72.13 ± 0.23 | 83.17 ± 0.11 |
20 mg/mL | 71.88 ± 0.01 | 82.99 ± 0.08 |
30 mg/mL | 71.91 ± 0.12 | 83.10 ± 0.03 |
50 mg/mL | 71.93 ± 0.08 | 83.20 ± 0.02 |
100 mg/mL | 71.85 ± 0.01 | 83.01 ± 0.01 |
At a concentration of 100 mg/mL, two transitions are clearly visible, correlating to the unfolding of the CH2 domain at an average Tmax,1 of 71.85 °C, and the combined Fab and CH3 domain at an average Tmax,2 of 83.01 °C [2]. No significant change in stability is observed as protein concentration is decreased and excellent reproducibility across calorimeters is observed. At 20 mg/mL Herceptin Trastuzumab, the magnitude of the CH2 unfolding transition is significantly diminished, however excellent reproducibility is observed across triplicate samples (Figure 3.). Below 20 mg/mL, analysis of the smaller CH2 unfolding domain becomes more challenging and increased variability in the detected Tmax,1 is observed. Larger unfolding events, such as the combined Fab and CH3 domains (Tmax,2) are more readily detected, even at concentrations as low as 5 mg/mL. As with all DSC instruments, sharper, narrower peaks provide the highest reproducibility. Limit of detection is sample dependent and can vary depending on the complexity of the protein and the solution environment.
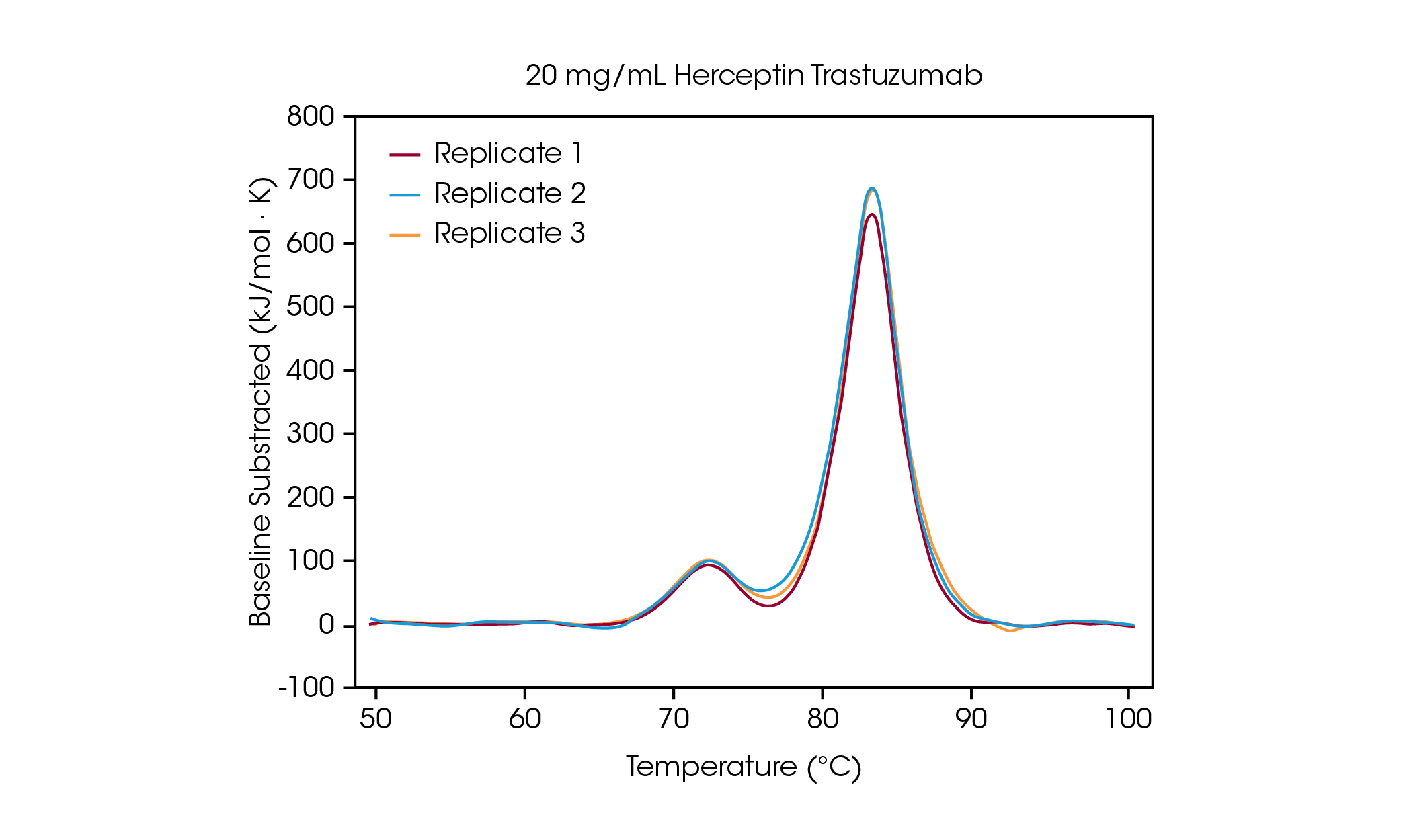
Investigation of Concentration Dependence on Thermal Stability
The TA Instruments RS-DSC is uniquely designed to handle high concentration biologic drug samples, with a specialized focus on antibody drugs and antibody drug conjugates. With the growing success of antibody therapeutics, the pharmaceutical industry has increased interest in high concentration dosage forms that enable subcutaneous and ocular drug delivery. As such, concentrations of 50 – 150 mg/mL antibody are common, with some being as high as 200+ mg/mL [3]. Formulating proteins at high concentrations can increase susceptibility to physical instability [4, 5]. Conversely, in some cases studies have shown enhancement in thermal stability at increased concentrations [6]. Thus, understanding of thermal unfolding and response to the solution environment at the formulation concentration of interest is a critical metric for mitigating drug product liability.
The TA Instruments RS-DSC is designed to contain protein samples in disposable microfluidic chips. Traditional microcalorimetric instrumentation contains a fixed sample cell that requires cleaning between experiments. High concentration samples that are susceptible to precipitation or gelation during the unfolding process can be difficult to clean at best or may damage the cell at worst. In addition to improving operational ease and eliminating time-consuming cleaning protocols, the disposable microfluidic chips used in the TA Instruments RS-DSC enable the risk-free testing of high concentration protein samples.
To demonstrate the ability to test high concentration protein samples and illustrate the importance of testing at the desired formulation concentration, we evaluated chicken egg white lysozyme from 30 – 330 mg/mL in glycine buffer (Figure 4 and Table 2). With a simple single transition thermogram at low concentrations (~1 mg/ mL), lysozyme is commonly used as a reference test sample for DSC. Through evaluation of protein concentrations up to 100-fold higher, we observed a concentration dependence on lysozyme stability.
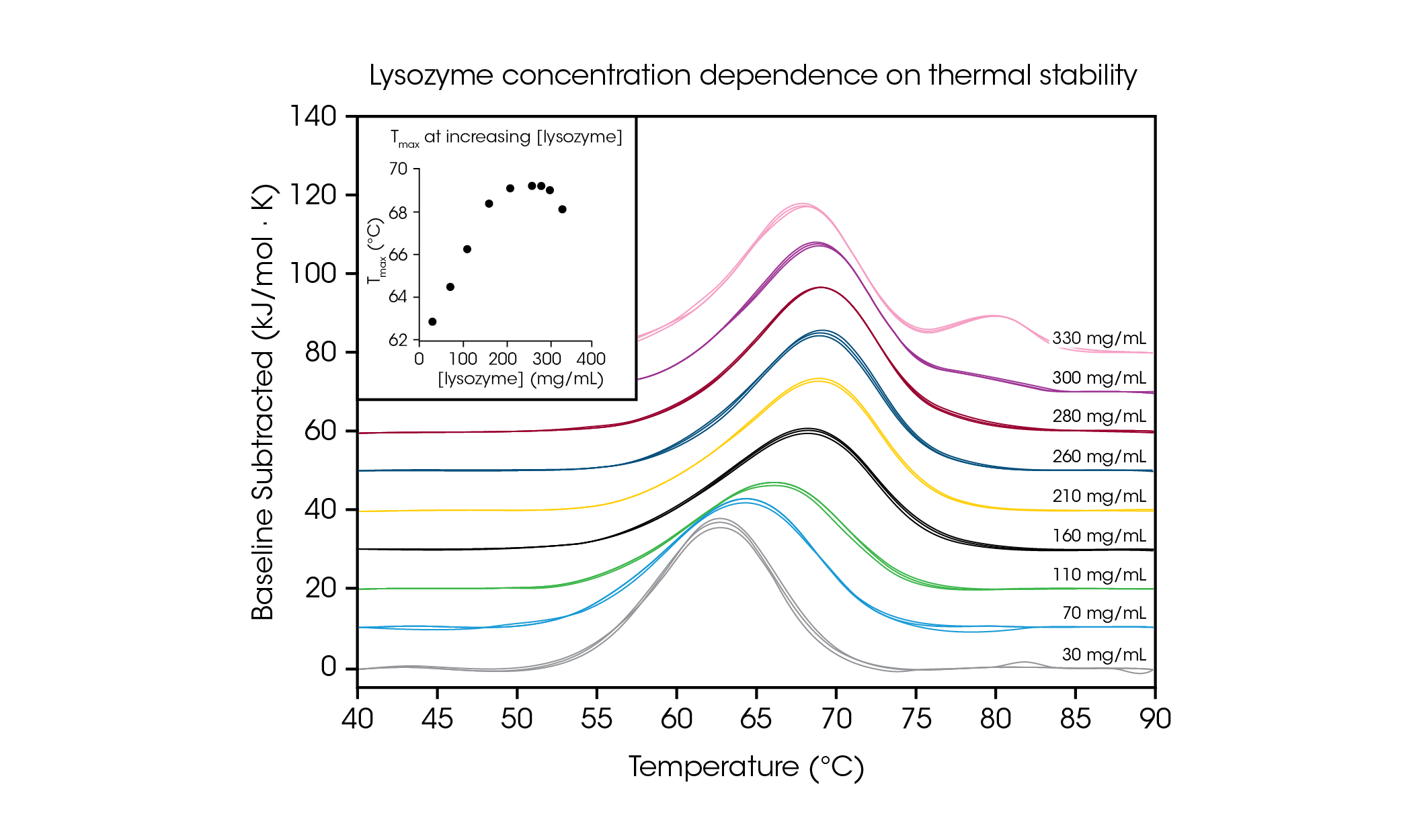
Table 2. Transition temperatures and reproducibility across the screen of lysozyme concentration dependence. Mean ± SD, n = 3.
Conc. | Tmax (°C) |
---|---|
330 mg/mL | 68.14 ± 0.05 |
300 mg/mL | 69.00 ± 0.04 |
280 mg/mL | 69.22 ± 0.04 |
260 mg/mL | 69.19 ± 0.09 |
210 mg/mL | 69.08 ± 0.06 |
160 mg/mL | 68.39 ± 0.04 |
110 mg/mL | 66.27 ± 0.09 |
70 mg/mL | 64.68 ± 0.05 |
30 mg/mL | 62.81 ± 0.06 |
At the lowest tested concentration, 30 mg/mL, the Tmax of lysozyme was measured to be 62.81 °C. As concentration increased up to 160 mg/mL, stability increased linearly (see Figure 4 inset) to a Tmax of 68.39 °C. From 210 – 280 mg/mL, the thermal stability plateaued at a Tmax of ~ 69.2 °C. Interestingly, at a concentration of 300 mg/mL, the presence of a shoulder at the tail of the main transition is beginning to be observed around 80 °C and the overall stability of the main transition decreases slightly with a Tmax of 69.00 °C. At the highest concentration of 330 mg/mL, the thermal stability of the main transition Tmax,1 decreases further to 68.14 °C and an additional peak is observed with a Tmax,2 of 80.28 ± 0.04 °C. With the capability for high concentration testing, the TA Instruments RS-DSC allows for the observation of the formation of higher order structure(s) at lysozyme concentrations greater than ~300 mg/mL. In addition to demonstrating the ability to acquire high quality data at high protein concentrations, this study demonstrates the importance of testing biologic drug products at the concentration of interest, as dilution may alter the observed stability of the protein.
Conclusions
Traditional single-sample DSC technology is highly accurate but requires large sample volumes and can be a time-consuming technique in the drug development process. The TA Instruments RS-DSC enables higher-throughput thermal stability testing through simultaneous analysis of up to 24 samples. An easier workflow, increased rate of data acquisition through parallel testing, and faster analysis through automated peak detection algorithms significantly improve time to results. Herein, we demonstrate accurate reproducible analysis of complex-multiple transition antibody samples down to 20 mg/mL. The faster scan rate of 2 °C/min results in higher amplitude signals, which can aid in analysis of samples at or near the limit of detection. Studying high concentration lysozyme solutions up to 330 mg/mL reveals unexpected concentration dependence on thermal stability and highlights the importance of evaluating the drug-product concentration of interest. Overall, the TA Instruments RS-DSC provides a new platform for characterizing biotherapeutic stability — an important variable in understanding resilience to thermal stress, product quality, and supporting regulatory approval.
References
- “Standard Practice for Calibration of Fixed-Cell Differential Scanning Calorimeters,” ASTM E2603-15, 2023.
- K. J. Arlotta, A. V. Gandhi, H.-N. Chen, C. S. Nervig, J. F. Carpenter and S. C. Owen, “In-Depth Comparison of Lysine-Based Antibody-Drug Conjugates Prepared on Solid Support Versus in Solution,” Antibodies, vol. 7, p. 6, 2018.
- R. G. Strickley and W. J. Lambert, “A review of formulations of commercially available antibodies,” Journal of Pharmaceutical Sciences, vol. 110, pp. 2590-2608, 2021.
- J. Zarzar, T. Khan, M. Bhagawati, B. Weiche, J. Syndow-Andersen and S. Alavattam, “High concentration formulation developability approaches and considerations,” MAbs, vol. 15, pp. 1-13, 2023.
- S. J. Shire, Z. Shahrokh and J. Liu, “Challenges in the development of high protein concentration formulations,” Journal of Pharmaceutical Sciences, vol. 93, pp. 1390-1402, 2004.
- C. Zhang, J. W. Bye, L. H. Lui, H. Zhang, J. Hales, S. Brocchini, R. A. Curtis and P. A. Dalby, “Enhanced Thermal Stability and Reduced Aggregation in an Antibody Fab Fragment at Elevated Concentrations,” Molecular Pharmaceutics, vol. 20, pp. 2650-2661, 2023.
Click here to download the printable version of this application note.