Electrolyte Researchers Work to Creatively Solve Energy-Related Challenges
The Amanchukwu Group at the University of Chicago charts new territory with TA Instruments and Waters technology in the design and synthesis of novel electrolyte media for bleeding edge applications in batteries and electrocatalysis
Technology: Waters™ TA Instruments™ Thermogravimetric Analyzer, Differential Scanning Calorimeter, Discovery Hybrid Rheometer (DHR), TAM IV Calorimeter
Electrolyte Research at the University of Chicago
The Amanchukwu Laboratory in the Pritzker School of Molecular Engineering at University of Chicago works to creatively solve energy-related challenges, particularly energy storage and electrocatalysis. The group focuses on the design and synthesis of novel solid state and liquid electrolyte media, as well as the study of electrolyte instability and ionic transport phenomena for applications in batteries and electrocatalysis.
Within energy storage and electrocatalytic devices, electrolytes are a vital component that support ionic and molecular transport. Borrowing concepts from chemistry and tools from biology, the laboratory’s scientists aim to control interfacial phenomena at the electrode:electrolyte interface and understand degradation mechanisms. The team uses nuclear magnetic resonance (NMR) and molecular dynamics (MD) to study ionic transport behavior and ion solvation environment, respectively. The Amanchukwu group also collaborates with Argonne National Laboratory, a U.S. Department of Energy multidisciplinary science and engineering research center, to use advanced characterization tools for in situ and operando understanding of energy devices.
Working with technology from TA Instruments, a subsidiary of Waters Corporation, the Amanchukwu Laboratory is charting new territory in the design and synthesis of novel electrolyte media for bleeding edge applications in batteries and electrocatalysis.
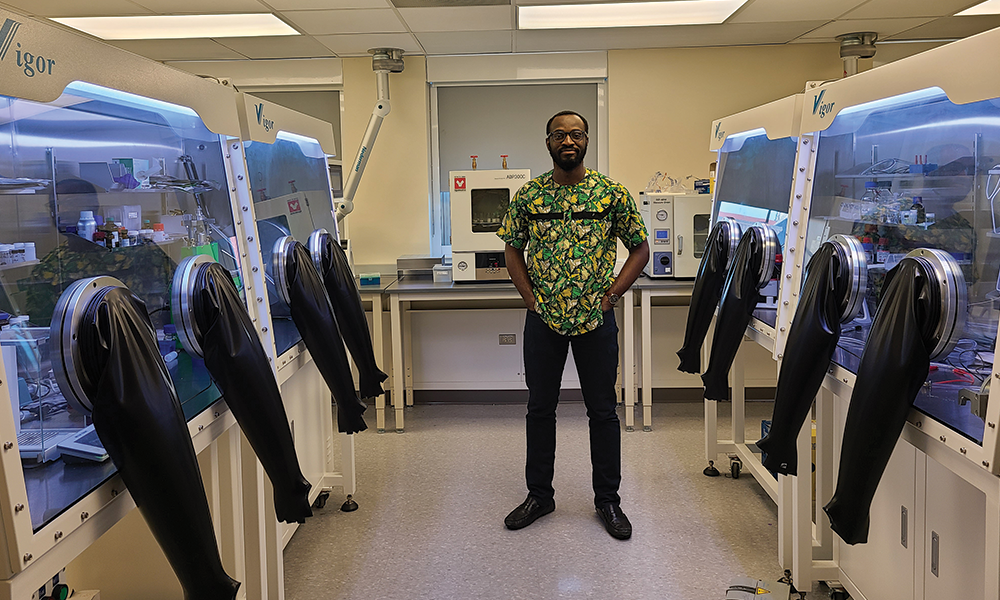
Dr. Amanchukwu and his team are pushing the boundaries in the synthesis of new small molecules, polymers, and hybrid solid-state electrolytes that can be used in next-generation batteries.
Working with Waters
After learning more about how other researchers were using thermal analysis, rheology, and microcalorimetry in different applications, Chibueze Amanchukwu, Principal Investigator of the Amanchukwu Laboratory and Assistant Professor at the University of Chicago, investigated how he could apply the same technology to his research in the design and synthesis of novel electrolyte media.
While Dr. Amanchukwu did look at other suppliers, only TA Instruments and Waters had the scientific expertise with the instrumentation that his team needed to achieve their goals. He explains:
“We are using this technology in an entirely new application, and no other instrument on the market was suitable. Fortunately, TA Instruments and Waters knew how to help us because they have great experience. We had multiple meetings and email exchanges with the TA Instruments and Waters teams, and they were able to help us. Plus, if we have any issues, we can speak to them, and they get back to us with a solution very quickly.”
A lot of current work with electrolytes could be called a “Wild West” approach, where you mix stuff and see if it works. We’re trying to bring more science to that process. We want to understand what it takes to analyze electrolyte properties, and then design electrolytes with the intent that changing a property will influence the battery in specific ways.”
– Dr. Chibueze Amanchukwu
Principal Investigator of Amanchukwu Laboratory and Assistant Professor at the University of Chicago
Design of the Next Generation of Batteries
The availability of energy-dense and compact batteries has galvanized interest in the electrification of transport and further integration of renewables in the energy grid. Rechargeable lithium-ion (Li-Ion) batteries are the batteries of choice today because they have the highest energy densities. However, their energy densities and cost are currently insufficient to keep pace with the insatiable demand for longer range electric vehicles and new electronic devices, as well as reduced carbon footprint.
Many next-generation batteries are limited by electrolyte selection, because high ionic conductivity and poor electrochemical stability are typically observed in most electrolytes. To address this issue, the Amanchukwu Laboratory at the University of Chicago is researching the design of new molecules with high ionic conductivity and high electrochemical stability for the rational design of next-generation batteries.1
The laboratory’s research focuses on the topics of:
- New electrolyte synthesis
- Electrode: electrolyte interface characterization
- High energy density batteries
- Selective and efficient electrocatalysis
As a newly appointed assistant professor at the University of Chicago, Dr. Amanchukwu is pushing boundaries in the synthesis of new small molecules, polymers, and hybrid solid state electrolytes that can be incorporated in next generation batteries (lithium metal, solid state batteries, and multivalent), and for carbon dioxide (CO2) electroreduction.2 The lab’s work strives to correlate bulk electrolyte structure (e.g., solvation) to electrochemical reactions (desired and undesired) that occur at the electrode:electrolyte interface. By understanding and controlling solvation effects in electrocatalytic media, Dr. Amanchukwu is also at the forefront of designing highly selective and efficient electrochemical reactors for CO2 conversion to valuable products. He describes the impact of this research:
“Electrolytes tend to be the forgotten parts of a battery. An ideal electrolyte is supposed to allow ions to move back and forth. But in real-life systems, they are often the main reason why batteries fail or don’t last as long as they should. It’s not well understood. We’re looking at bringing more science to electrolyte design. We are using molecular design strategies to develop electrolytes and predict what they would do in a battery cell.”
Those predictions would include reducing undesirable parasitic reactions, which are a major contributor to the degradation of the electrochemical performance in many battery chemistries.
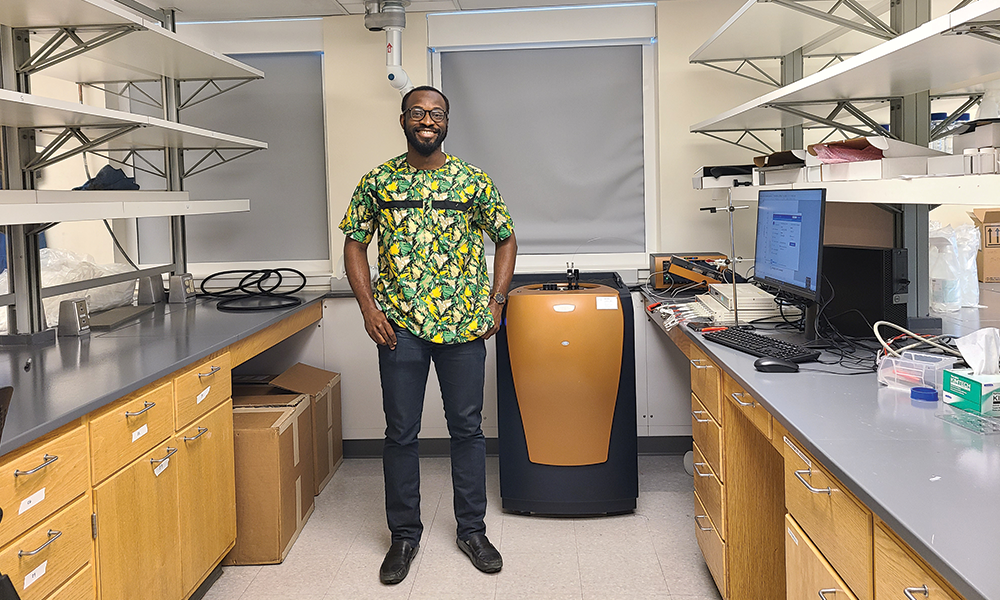
The TAM IV microcalorimeter system is used to measure the universal heat signal, and the quantitative thermodynamic and kinetic behavior of electrolytes.
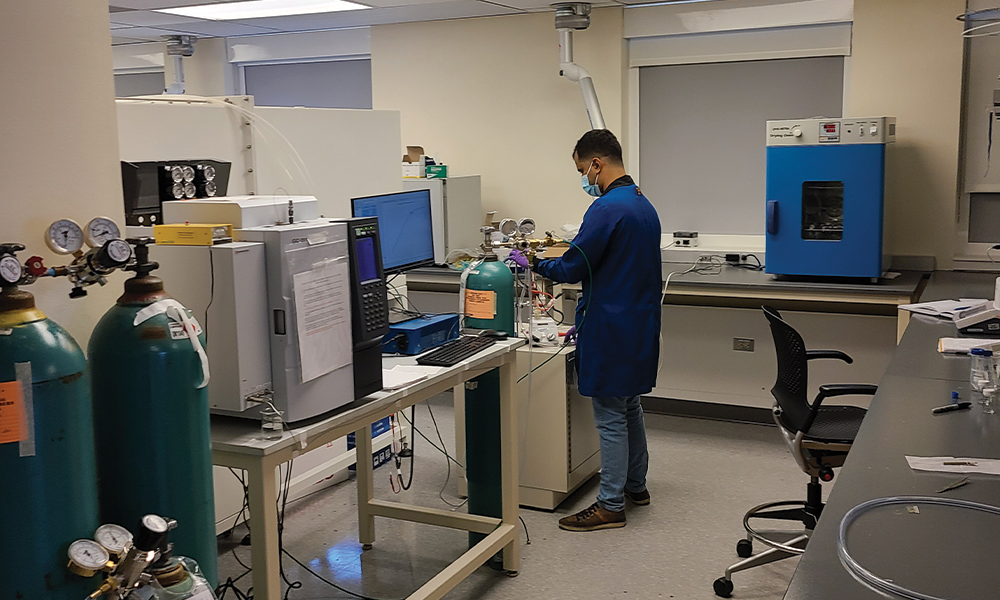
The Amanchukwu Laboratory’s work strives to correlate bulk electrolyte structure to electrochemical reactions that occur at the electrode:electrolyte interface.
These side reactions between the electrode materials and the nonaqueous electrolytes are a key factor when designing next-generation batteries for practical applications. Dr. Amanchukwu comments:
“Often, if the electrolyte is unstable, it will create these undesirable reactions (known as parasitic reactions) upon contact with that battery electrode. So, they are intrinsically linked. Part of the definition of a good electrolyte is how well it prevents these parasitic reactions.”
Dr. Amanchukwu believes that newly designed electrolytes coupled with an improved understanding of ion transport and electrochemical degradation will enable the fabrication of batteries with high energy density and longer cycle life. And that could have a large impact on the manufacturing quality of energy-dense and compact batteries in the future, and how those batteries are used. He explains:
“I think next-generation batteries can be at least 50 percent better. So that opens up a lot more applications. For example, those levels of improvements could make electric vehicles much more amenable for the general population to purchase. And there are so many ways these batteries could find applications that we haven’t even envisioned today.”
To meet these expansive goals, the Amanchukwu Laboratory is taking cues from another industry that found new applications unimaginable just a decade or two ago – pharmaceuticals.
“ It’s quite exciting to work with the TAM IV microcalorimeter system. No other instrument has the heat flow sensitivity we need for this research. We’ve discovered we can use microcalorimetry to gather a lot of information, and it’s extremely easy to use. But when we couple it with other techniques, it provides us with even more insight for our research. Another benefit is the ease of use – even for those with minimal or no training, it’s very simple for users to grasp the instrument quickly.”
– Dr. Chibueze Amanchukwu
Principal Investigator of Amanchukwu Laboratory and Assistant Professor at the University of Chicago
Inspiration from Biology
All chemical and biological processes are associated with heat production or heat consumption. Dr. Amanchukwu found inspiration for his own research from the way the pharmaceutical industry uses technologies – in this instance microcalorimetry – to measure the heat release or heat absorption in chemical processes.
That sparked the idea to investigate how the same instrumentation could be used to understand how electrolytes interface in different battery chemistries. Among its many tools, the Amanchukwu Laboratory uses microcalorimetry for kinetic and thermodynamic quantification, and NMR (solid and solution state) for chemical specificity.3 Dr. Amanchukwu explains:
“The pharma and drug discovery worlds are already using this technology to measure protein or drug binding interactions, or to measure heat released from cell behavior. So, I started to wonder if we could do the same thing? The chemical processes are agnostic to whether they are being used in a battery or in material science because the binding interactions are universal. We actively look at other industries and see what helps them answer the questions they’re interested in, and then turn it around to see if the same tools can help us answer similar questions in batteries, electrolytes and material science.”
Working with Waters, scientists at the Amanchukwu Laboratory began using the TA Instruments TAM IV microcalorimeter system to directly measure the universal heat signal and, therefore, the quantitative thermodynamic and kinetic behavior of electrolytes. The heat flow sensitivity and long-term temperature stability of the TAM IV enabled Dr. Amanchukwu and his team to measure many processes that were undetectable by other techniques.
Those other techniques include Raman spectroscopy, infrared spectroscopy, and mass spectrometry – all supported by Waters. Dr. Amanchukwu is particularly interested in testing new combinations of TA Instruments and Waters technology, and he sees the possibilities for his team’s bleeding edge electrolyte research. He explains:
“Microcalorimetry is amazing because it gives us a quantitative understanding, but we can also couple this technology with spectroscopic techniques that can show how the interaction between two functional groups is responsible for the heat release or the heat absorption that we are seeing in the calorimeter. These multi-modal approaches give us the benefit of both worlds.”
Furthermore, Dr. Amanchukwu sees potential in the high- throughput screening tools typically used in biological-based research that could also be deployed for rapid screening of electrolyte properties. Without current budget restrictions, he believes this advanced technology – which is also well known and used by Waters customers in pharma and life sciences – could significantly impact electrolyte research.
“ We have access to instruments in other departments within the University, which is very helpful. As a growing laboratory, we have great ambition, and would love to include a Waters mass spectrometer of our own to add advanced screening to our experiments. This screening approach is used in the pharmaceutical industry, and the principles are the same. If you’re trying to discover a drug to cure whatever disease is of interest, there are trillions of molecules that you can possibly try. How do you feasibly approach that task? That’s the same challenge that we face in batteries.”
– Dr. Chibueze Amanchukwu
Principal Investigator of Amanchukwu Laboratory and Assistant Professor at the University of Chicago
Data Science
Dr. Amanchukwu and his team also see the potential of artificial intelligence (AI) and machine learning (ML) tools to generate new ideas for electrolyte compounds for next generation batteries, as well as investigate novel solvation behavior for CO2 electrocatalysis.
By collaborating with data science and computational experts, the team plans to use natural language processing (NLP) tools to develop a database of experimentally measured electrolyte properties. ML models can then be made to correlate accessible electrolyte features (e.g. molecular structure) to properties such as conductivity and stability.4 Dr. Amanchukwu explains:
“What we are doing right now is more manual. That’s why we’re interested in the impact of data science on this research. You can work on electrolytes for decades and not have any success, or you can use a data science approach to narrow down the possibilities that can feasibly be investigated in the lab.
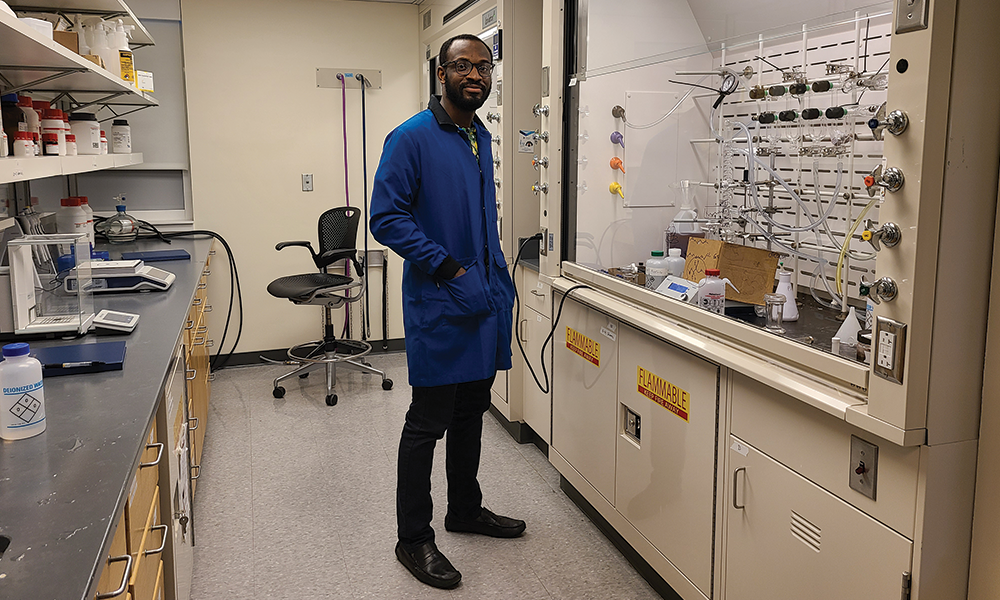
Dr. Amanchukwu found inspiration for his research from the way that the pharmaceutical industry uses microcalorimetry to measure heat release and heat absorption in chemical processes.
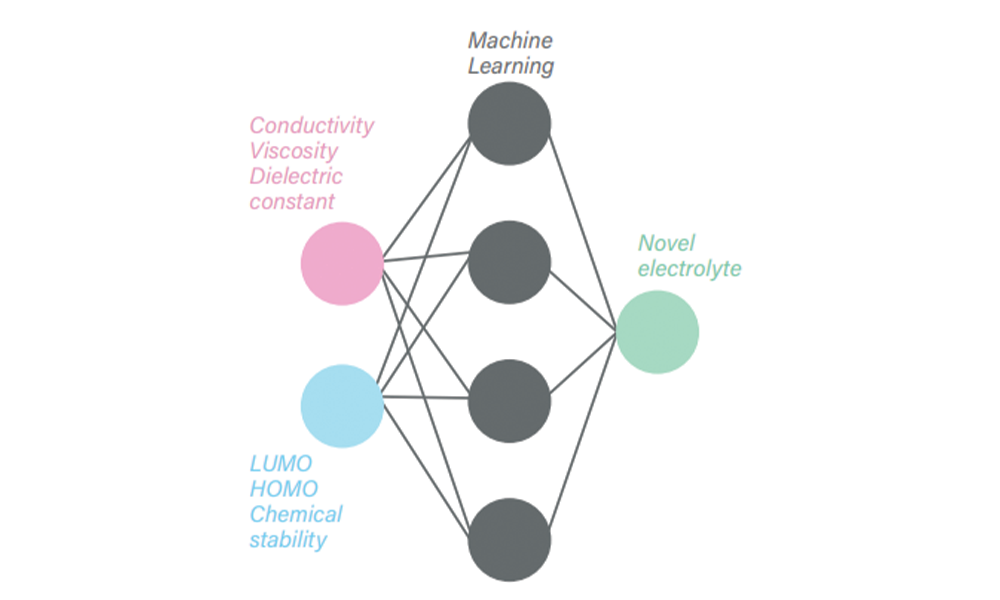
Figure 1. Data science could eventually be used to get predictive knowledge on how electrolytes could behave in battery chemistry with a better understanding of the heat profile.
“ We know that we can make milligram quantities of these electrolytes, but can we make grams and kilograms? One of the exciting parts of our work is talking to companies to get them interested in the compounds we make. If we can show that they work and they are useful, those companies can scale it up and use them in the manufacturing process.”
– Dr. Chibueze Amanchukwu
Principal Investigator of Amanchukwu Laboratory and Assistant Professor at the University of Chicago
Real World Applications
The potential of energy-dense and compact batteries has attracted interest from other parties, particularly for addressing societal issues related to climate change. The Amanchukwu Laboratory’s research has significant implications for the design of next-generation batteries. The group is interested in working with Argonne National Laboratory to make pilot-scale quantities of electrolyte solvents, which a private company could scale up for use in battery manufacturing.
The real-world applications of the Amanchukwu Laboratory’s research are extensive, and the group eventually plans to work with the University of Chicago’s Polsky Center for Entrepreneurship and Innovation to find companies interested in further developing the results of their research.
“ We work hand in hand with the Polsky Center on some of the novel compounds that we’ve developed. One thing I love about working on electrolytes is that it’s really translatable from academia to the commercial realm. For liquid electrolytes, it doesn’t require any change in manufacturing capability because you just replace the liquid that the company is currently using in their battery with our new liquid electrolytes.”
– Dr. Chibueze Amanchukwu
Principal Investigator of Amanchukwu Laboratory and Assistant Professor at the University of Chicago
Next Steps
As a newly established laboratory, the Amanchukwu group is looking at the long-term potential in its research. In five years or so, Dr. Amanchukwu sees the lab achieving its goal of developing next generation energy-dense batteries that will be enabled by novel electrolyte design strategies. However, he also wants to develop methodology to predict how an electrolyte will behave before testing, as well as insights into why these compounds behave in the way they do. He describes one such possibility:5
“One of our ideas is creating novel electrolyte compounds that are modular. So, if I have maybe three building blocks, I can switch those building blocks based on what the chemistry demands. This modular approach is exciting because it gives us a wide range of materials to study, but also allows us to adapt on the fly. When a new battery chemistry is created and we know that it needs a specific functionality, then we can mix and match in these new molecular designs by arranging those blocks and altering how they attach to one another.“
The bigger picture, however, is how the Amanchukwu Laboratory’s bleeding edge research could impact how energy is produced, delivered, and consumed. Improvements in battery life, for example, have long-range implications for climate change and its impact on societal and natural resources. That could lead to improvements that affect health, infrastructure, and transportation systems, in addition to energy supplies.
“ The engineering point of view definitely is a factor in how we design our projects. We also want to keep in mind how we can scale to really address societal challenges. In the end, we’re trying to creatively solve our energy-related challenges, so we can solve the problems associated with climate change.”
– Dr. Chibueze Amanchukwu
Principal Investigator of Amanchukwu Laboratory and Assistant Professor at the University of Chicago
References
- Amanchukwu, C.V.; Yu, Z.; Kong, X.; Qin, J.; Cui, Y.; Bao, Z. A New Class of Ionically Conducting Fluorinated Ether Electrolytes with High Electrochemical Stability. J. Am. Chem. Soc. 2020, 142, 16, 7393–7403.
- Yu, Z.; Wang, H.; Kong, X. et al. Molecular Design for Electrolyte Solvents Enabling Energy-dense and Long-cycling Lithium metal Batteries. Nat. Energy. 2020, 5, 526–533. https://doi.org/10.1038/s41560-020-0634-5.
- Gunnarsdóttir, A.B.; Amanchukwu, C.V.; Menkin, S.; Grey, C.P. Noninvasive In Situ NMR Study of “Dead Lithium” Formation and Lithium Corrosion in Full-Cell Lithium Metal Batteries. J. Am. Chem. Soc. 2020, 142, 49, 20814–20827.
- Amanchukwu, C.V. The Electrolyte Frontier: A Manifesto. Joule, 2020, 4, 1–5.
- Ma, P.; Mirmira, P.; Amanchukwu, C.V. Effect of Building Block Connectivity and Ion Solvation on Electrochemical Stability and Ionic Conductivity in Novel Fluoroether Electrolytes. ACS Central Science 2021 7 (7), 1232–1244.