How to Optimize 3D Printing Materials Through Thermal Analysis
Jason Saienga | Gray Slough | Morgan Ulrich
May 30, 2022
3D printing, also known as additive manufacturing, is being embraced as a versatile manufacturing technique across diverse industries. 3D printing allows for rapid prototyping and print-on-demand solutions to avoid the potential waste associated with batch runs.
One of the reasons 3D printing is being widely adopted in manufacturing is its unique ability to create complex shapes. Many standard manufacturing methods are unable to create cavities and undercuts in a structure. An additive approach allows for creating unusual shapes with ease.
Today, 3D printing is expanding to include a range of materials, including biocompatible polymers and metals. 3D printing is even being used in areas such as healthcare for the customized printing of medical devices.1
In order to optimize materials for 3D printing, manufacturers need to pay careful consideration to the mechanical and thermal properties of the final material. While 3D printed components tend to be lightweight and the right combination of polymer components can have a similar tensile strength to metals, overcoming the typically low mechanical and thermal properties of additive manufacturing parts is one of the biggest challenges.2
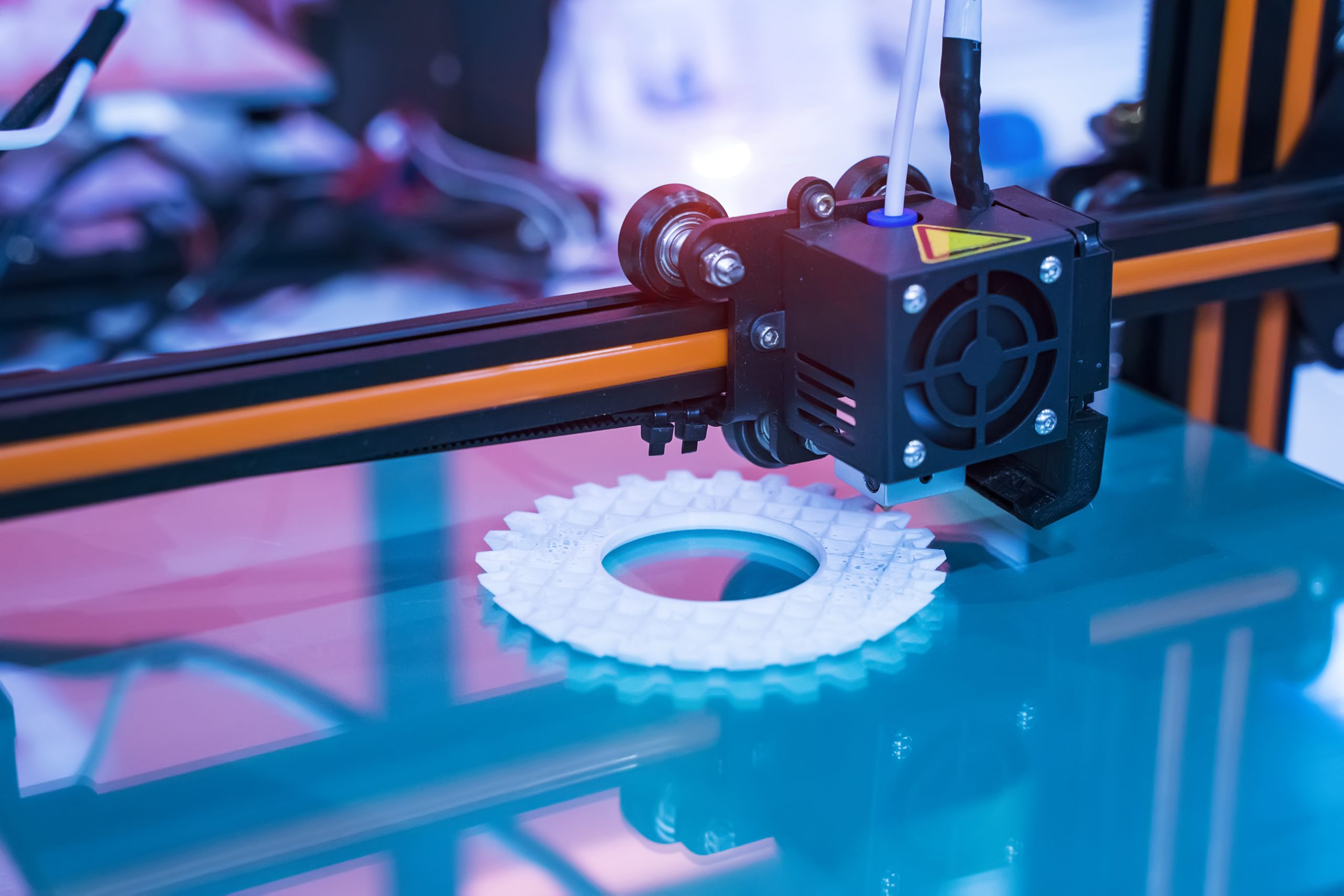
Process Optimization for 3D Printed Product Properties
Finding out how the extrusion process affects the final properties of the printed material is a highly active area of research. Automotive applications are some of the most demanding in terms of the tensile and thermal properties of the material.
Fortunately, there are a number of thermoplastic polymer matrices with carbon fiber, glass fiber and Kevlar available that are suitable for 3D printing parts and can achieve sufficient high performance for use in automotive applications.2
During the 3D printing process, the substrate to be printed is melted and then deposited in layers to create the final object. There are multiple parameters that can be optimized in the process, such as the bed and nozzle temperatures for the polymer and the cure time between layers.
There are a number of methods for 3D printing, including selective laser sintering, bio-printing and fused deposition modeling. Fused deposition modeling is the most common method.
The glass transition temperature is necessary information for choosing the right temperature to extrude amorphous polymers. For semicrystalline polymers, the melting temperature is the value of interest. The degree of crystallinity strongly influences the mechanical properties of the polymer.
Many polymers are cured with UV light, which generates radicals in the polymer material that act as initiators for the cross-linking process in the production of the final polymer. A higher degree of cross-linking typically results in a more rigid and strong material. Material strength from cross-linking can be influenced by altering the length of time the sample is exposed to UV light.
Both the temperature and cure time affect the polymer’s molecular structure in the material and its properties. Therefore, to optimize these parameters and explore their effects on the final material, material designers use testing techniques that are sensitive to the details of polymer properties.
Thermal Analysis of 3D Printing Materials
The main thermal analysis tools for investigating the influence of the extrusion process on the properties of the final material include thermogravimetric analysis (TGA), differential scanning calorimetry (DSC), thermomechanical analysis (TMA) and dynamic mechanical analysis (DMA).3 Each technique provides complimentary information that can be combined to give deeper understanding of the printing material’s properties.
Thermogravimetric analysis measures the magnitude and rate of change of the weight of a material as a function of temperature or time. TGA is very useful for characterizing the influence of extrusion as many materials, when heated, can undergo oxidation or decomposition, which results in weight changes.4 Thermogravimetric analysis is one of the best methods to determine whether the sample undergoes degradation under the conditions it will face in the extrusion process.
Differential scanning calorimetry is a powerful technique to measure the exothermic and endothermic transitions in a material as a function of temperature – common points of interest for extrusion processing include the glass transition temperature, melt temperature, and the heat capacity of the material.
Differential scanning calorimetry and thermal gravimetric analysis are a powerful and complimentary combination of techniques when used to understand the effects of extrusion. Together the techniques can be used to profile the thermal properties of the polymer at the extrusion temperature.3
Thermomechanical analysis , which measure coefficient of thermal expansion (CTE) and glass transitions temperatures, is another complementary technique. As the glass transition temperature is dependent on the thermal history of the material, thermomechanical analysis is a good way of checking that the extrusion process will not introduce any unwanted mechanical behavior into the finished object. Also, reinforced materials may show anisotropy in the CTE depending upon the measurement orientation relative to the fiber direction.3
Dynamic mechanical analysis is also widely used in materials engineering for the analysis of polymer composites as it can uncover information on the behavior of a material under dynamic loading conditions.5 DMA is especially useful for characterizing finished 3D printed parts, reflecting how different formulations and processing methods affect end-use performance.
Choosing the Right 3D Printing Thermal Analysis Technique
Most 3D printing manufacturing lines rely on a combination of the techniques mentioned above. As the world leader in Thermal Analysis, TA Instruments is the instrumentation provider of choice for additive manufacturers around the world.
We help users across various industries find the right instrument and method for their unique 3D printing challenges and goals. We offer a range of industry-leading thermal analysis instruments that are designed to be easy-to-use and highly efficient. TA Instruments’ comprehensive line of thermal analysis products has all the necessary devices to completely characterize the thermal and mechanical properties of your substrates.
Contact our experts today to find out how the TA Instruments range of thermal analysis and mechanical analysis instruments can improve the quality of your 3D printed products.
References:
- Trenfield, S. J., Awad, A., Madla, C. M., Hatton, G. B., Goyanes, A., Gaisford, S., Basit, A. W., Trenfield, S. J., Awad, A., Madla, C. M., & Hatton, G. B. (2019). Shaping the future: recent advances of 3D printing in drug delivery and healthcare. Expert Opinion on Drug Delivery, 16(10), 1081–1094. https://doi.org/10.1080/17425247.2019.1660318
- Mohammadizadeh, M., & Fidan, I. (2019). Thermal Analysis of 3D Printed Continous Fiber Reinforced Thermoplastic Polymers for Automotive Applications. Solid Freeform Fabrication 2019: Proceedings of the 30th Annual International Solid Freeform Fabrication Symposium – An Additive Manufacturing Conference, 899–906. https://utw10945.utweb.utexas.edu/sites/default/files/2019/078%20Thermal%20Analysis%20of%203D%20Printed%20Continuous%20Fiber%20Re.pdf
- Billah, K. M., Lorenzana, F. A. R., Martinez, N. L., Chacon, S., Wicker, R. B., & Espalin, D. (2019). Thermal Analysis of Thermoplastic Materials Filled with Chopped Fiber for Large Area 3D Printing. Solid Freeform Fabrication 2019: Proceedings of the 30th Annual International Solid Freeform Fabrication Symposium – An Additive Manufacturing Conference, 892–898. https://utw10945.utweb.utexas.edu/sites/default/files/2019/077%20Thermal%20Analysis%20of%20Thermoplastic%20Materials%20Filled.pdf
- TA Instruments (2022) 3D Printing Webinar, https://www.tainstruments.com/3-d-printing-and-additive-manufacturing-process-optimization-a-thermal-approach/, accessed May 2022
- Saba, N., Jawaid, M., Alothman, O. Y., & Paridah, M. T. (2016). A review on dynamic mechanical properties of natural fibre reinforced polymer composites. Construction and Building Materials, 106, 149–159. https://doi.org/10.1016/j.conbuildmat.2015.12.075