Keywords: powder rheology, lactose, flowability, shear, pharmaceutical, excipient, drug formulation, tableting
RH123
Abstract
Powder flow measurements are increasingly important in pre-formulation and formulation stages as pharmaceutical developers continue to push towards quality-by-design approaches. TA Instruments Powder Rheology Accessory includes two cells for powder measurements: the Flow Cell and the Shear Cell. In this note, the processing behavior of two lactose excipients is studied by measuring shear and flowability of milled and spray-dried lactose. Milled lactose was found to have higher cohesion, yield strength, confined and unconfined flow energies when compared to spray-dried lactose. Additionally, milled lactose flow energy exhibited a dependence on impeller tip speed and more variability than spray-dried lactose. The multiple powder rheology measurements presented indicate better processability with spray-dried lactose than with milled lactose.
Introduction
Drug formulators must choose excipients that meet processing and performance needs such as flowability, cohesion, stability, and compressibility. Lactose is a commonly used excipient that typically undergoes a two-stage manufacturing process. It is first milled to a powder, creating particles with irregular size and morphology. To achieve characteristics desirable for different products and formulations, a secondary process is applied. Spray-drying is a secondary process that yields spherical particles of more uniform size, allowing the lactose to flow more easily [1]. This size control and flowability makes it desirable for dry granulation and has also shown advantages in wet granulation tableting.
With the increased push towards quality-by-design (QbD) approaches for developing pharmaceutical products, especially by regulatory authorities around the world, powder flow measurements are a critical part of the analytical workflow in the pre-formulation and formulation stages [2]. Powder rheology enables the quantification of properties in the lab to optimize formulations before scale-up. The flowability of free-flowing powder combined with the shear and compressibility of consolidated powder provide important key insights to not only formulators, but also suppliers as these measurements can guide hopper design. This study uses powder rheology measurements to characterize milled and spray-dried lactose.
Experimental
Milled lactose (Loudwolf Industrial and Scientific) and spray-dried lactose (SpecializedRx) were used in this study. All measurements were performed using a TA Instruments rheometer and the Powder Rheology Accessory, with interchangeable shear and flow cells, shown in Figure 1. For these tests, the Discovery HR-30 rheometer was used at ambient room conditions of 21 °C and 50% relative humidity.
Application Benefits
- The processability of a powder pharmaceutical formulation is impacted by the choice of ingredients.
- Powder rheology is a valuable technique to evaluate ingredients or formulations for unexpected behavior during processing.
- The HR Powder Rheology Accessory includes both Flowability and Shear measurements, providing a complete picture of powder flow behavior and particle interactions.
- Insights into powder rheology enable users to optimize formulations to ensure production success, accelerating new product development.

Powder Flowability
The Powder Flow Cell was used to measure flowability by moving an impeller rotor through a powder bed in a helical path. Unconfined flow is measured as the impeller moves up through the powder and confined flow is measured as the impeller moves down through the powder. Prior to testing, the powders were loaded into the cup and conditioned by moving the impeller upwards and downwards through the powder at a tip speed of 100 mm/s, then trimmed, as shown in Figure 2. This procedure ensures uniformity between test samples and improves reproducibility of results. After conditioning, the impeller followed a helical path with a tip speed of 100 mm/sec, with one testing cycle used to measure confined and unconfined flow. This was repeated seven times for a total of seven measurement cycles to evaluate stability. Upon completion of these seven cycles, one cycle each was performed at 100, 70, 40, and 10 mm/sec to evaluate rate dependence. Normal force and torque were measured to determine flow energy and testing was repeated three times using fresh samples.
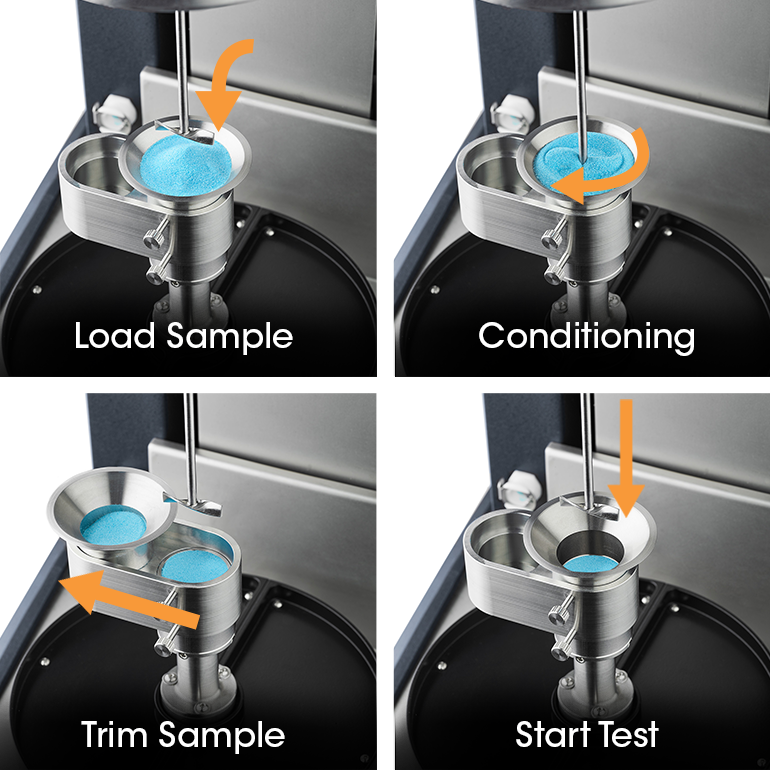
Powder Shear
Shear measurements were made using the Shear Cell accessory, shown in Figure 3, which has a serrated upper plate and cup to prevent slip when shearing the compacted powder. The lactose powders were loaded using the provided loading slide and funnel, then consolidated by applying a stress of 15 kPa. The sample was then trimmed to remove excess and level the surface for testing.
Testing was performed per ASTM D7891 [3], using a consolidation normal stress of 15 kPa. A pre-shear step was performed, maintaining the 15 kPa consolidation stress on the powder sample, and slowly rotating (angular velocity of 1*10-3 rad/sec) until the measured shear stress reached steady state. Next, the normal stress was reduced, and rotation applied until the powder showed an incipient yield, in form of a peak in shear stress. The pre-shear step was repeated with the same initial normal stress (15 kPa) to achieve consistent powder consolidation conditions, then followed with a shear measurement under a lower normal stress. A total of ten test cycles were completed under normal stresses from 12 kPa to 3 kPa.
Table 1. Multi-step powder shear test procedure
Cycle | Pre-Shear Stress (kPa) | Test Stress (kPa) |
---|---|---|
1 | 15 | 12 |
2 | 15 | 11 |
3 | 15 | 10 |
4 | 15 | 9 |
5 | 15 | 8 |
6 | 15 | 7 |
7 | 15 | 6 |
8 | 15 | 5 |
9 | 15 | 4 |
10 | 15 | 3 |
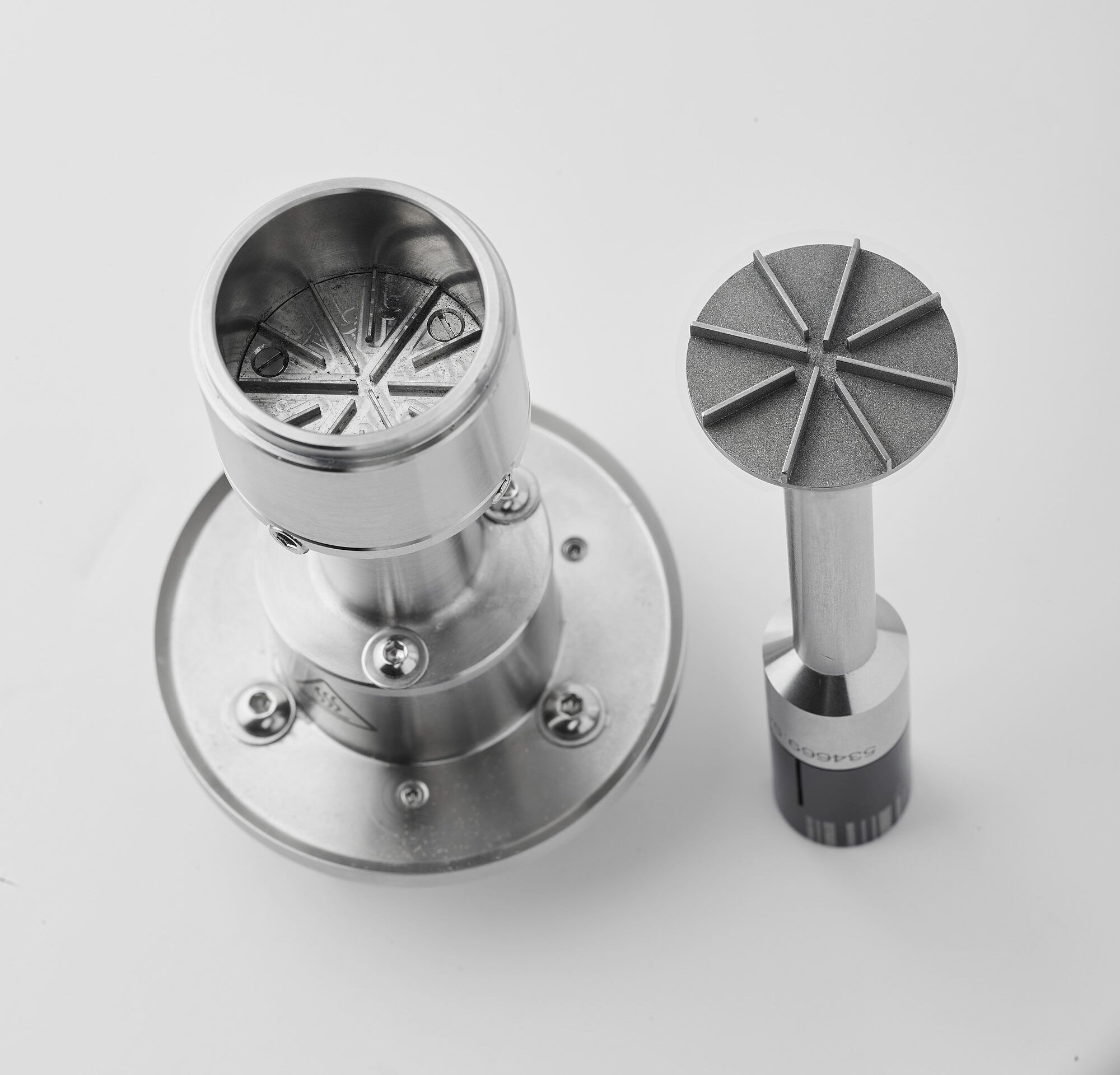
Results and Discussions
Powder Flowability
The flowability energy results for milled and spray-dried lactose are shown in Figure 4. The higher flow energy of the milled lactose has important implications in manufacturing and scale up processes. To flow the excipient into the industrial tableting mold, the milled lactose will require higher energy and other considerations to the critical process parameters (CPP).
Unconfined flow is sensitive to the particles’ tendency to interlock [4]. Milled lactose shows higher unconfined flow energy than spray-dried lactose due to different particle shapes. Milling breaks lactose into shards with rough surfaces and high aspect ratio, while the spray-drying process forms spherical particles that more easily slide past each other during flow, as seen in Figure 5. Both samples show reproducible results on the first cycle, with flow energy values within 1.5% for the 3 replicates. Spray-dried lactose shows very little change during repeated testing.
Milled lactose also exhibits a rate dependency not seen with the spray-dried particles. In Figure 6, the confined flow energy of the milled lactose decreases with increasing tip speed whereas the spray-dried lactose does not show a rate dependency. This is key information for a formulator during early stage drug development as a change in the excipient source can lead to significant differences in processability during scale up. If a formulator changes the excipient source from spray-dried to milled, they may see performance differences during low-speed processes such as flowing into a mold before pressing into a tablet. Furthermore, the dependency of the confined flow energy on the transfer speed can cause inconsistencies in the final dosing of the excipient resulting in a wider-than-acceptable spread of the critical quality attributes (CQA) of the final product.

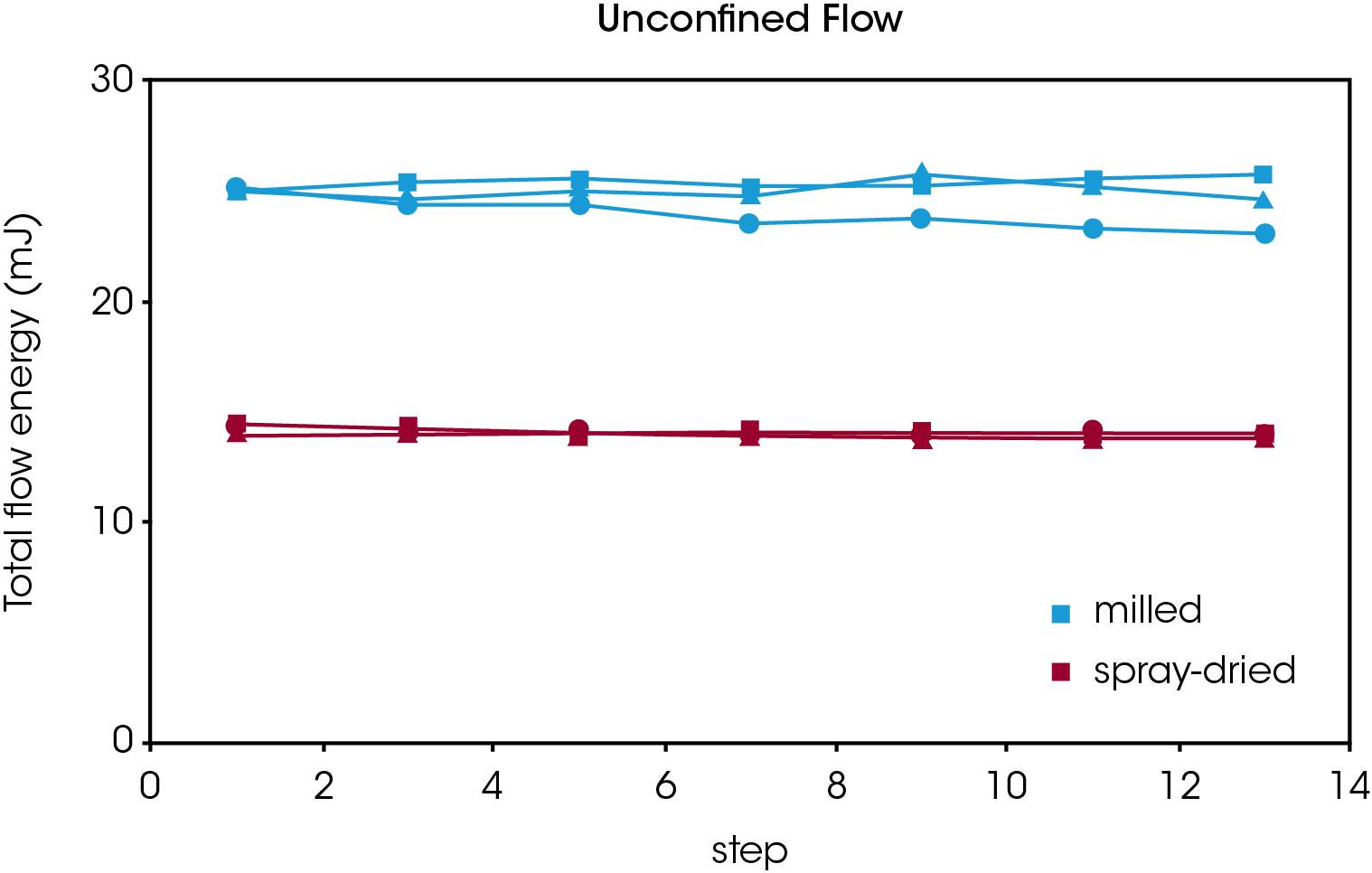

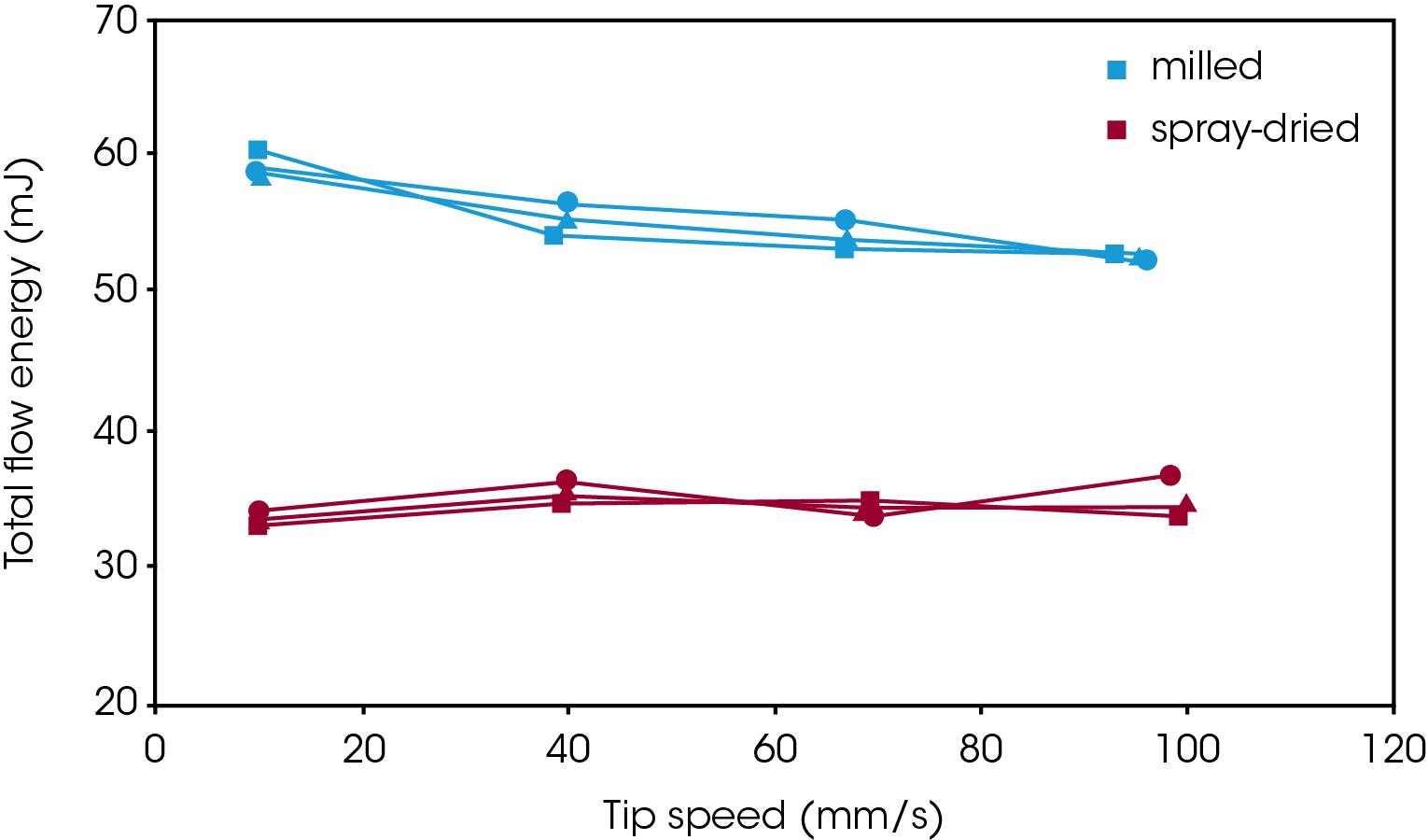
Powder Shear
Figure 7 and Table 2 show the shear results of spray-dried and milled lactose samples. TRIOS software was used to perform Yield Locus Analysis and calculate results as defined by ASTM D7891. Steady-state stress of all pre-shear steps is determined and averaged (circle symbol). For each shear step, the incipient yield points are identified and plotted (square symbols).
The yield locus line is a line of best fit drawn through the shear data points. The y-intercept indicates the cohesion between particles. A Mohr circle is drawn such that it passes through the graph origin and lies tangent to the yield locus line; the x-intercept of this circle indicates unconfined yield strength. A second Mohr circle is drawn such that it passes through the pre-shear average coordinates and lies tangent to the yield locus line. The greater x-intercept indicates major principal stress (maximum internal stress under consolidation).
Table 2. Shear results for milled and spray-dried lactose
Shear Results (kPa) | Milled Lactose | Spray-Dried Lactose |
---|---|---|
Cohesion | 1.01 | 0.10 |
Unconfined Yield Strength | 4.24 | 0.37 |
Major Principal Stress | 33.33 | 27.72 |
As shown in Figure 7 and Table 2, spray-dried lactose has negligible cohesion and shear strength, which is desirable for processing. Due to particle morphology, interactions occur between milled particles, resulting in higher cohesion and yield strength. This higher yield strength will impact the dispensing of the lactose powder as flow will not occur until the yield strength is overcome. To achieve uniform dispersion, the milled particles may also require longer mixing to break up interactions due to the higher cohesion, ultimately impacting the dispersion of the active pharmaceutical ingredient (API) into the excipient.
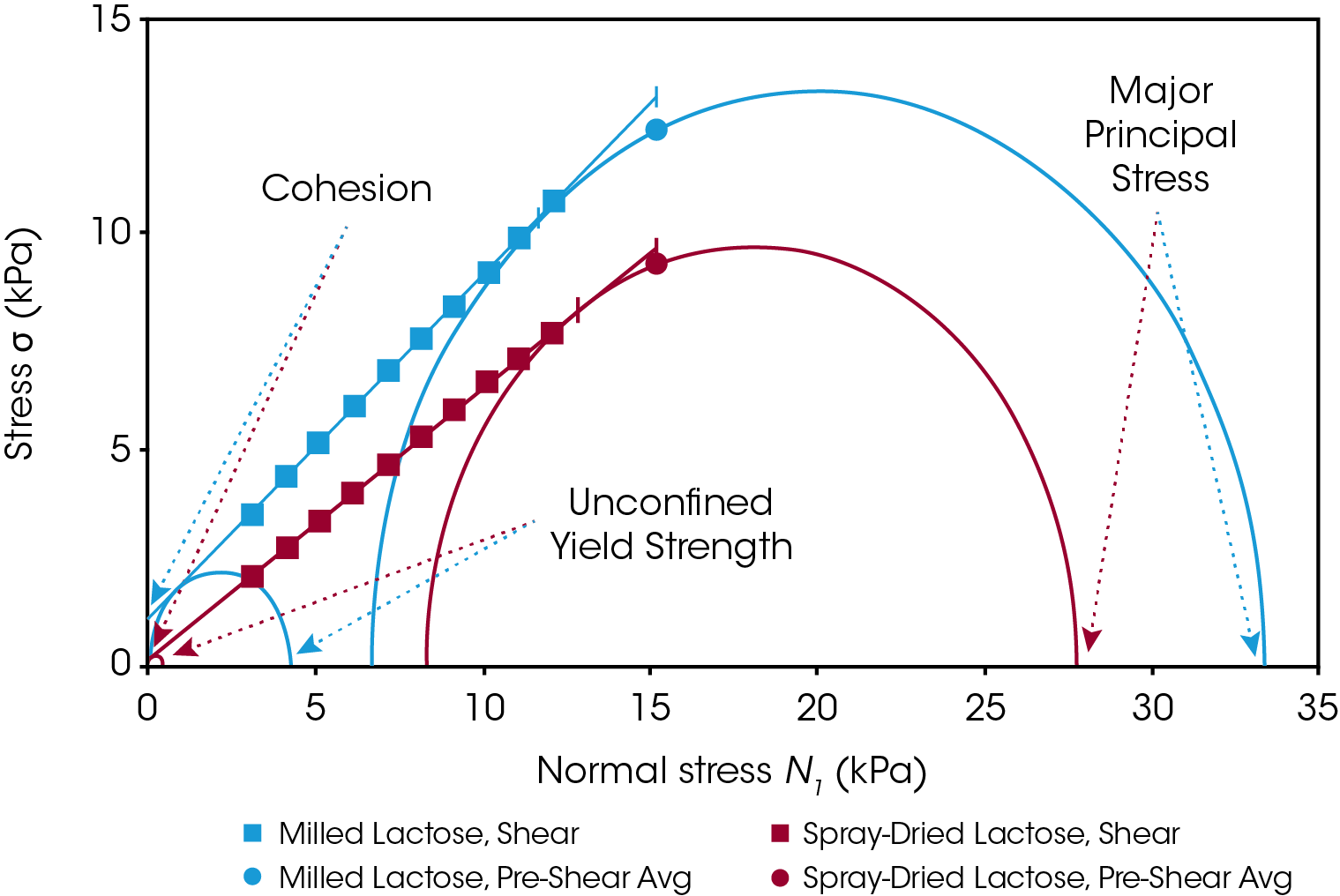
Conclusions
Flow properties of milled and spray-dried lactose were measured using the TA Instruments Powder Rheology Accessory. The Flow Cell was used to measure confined and unconfined flow, while the Shear Cell measured shear and cohesion of the powders. This information is critical as manufacturers increasingly use QBD approaches for drug development. The irregular morphology of the milled lactose results in higher flow energies, and a rate dependence not seen in spray-dried lactose. Spray-dried lactose, with lower flow energy, better flow stability, and negligible cohesion, has better processability than milled lactose. This information is important in understanding process development during the scale up stage and recognizing that changes to excipients impact transfer speed and CQA of the final product.
References
1. W. Huang, Y. Shi, C. Wang, K. Yu and Y. Li, “Using spray-dried lactose monohydrate in wet granulation method for a low-dose oral formulation of a paliperidone derivative,” Powder Technology, vol. 246, pp. 379-394, 2013.
2. K. Pramod, M. A. Tahir, N. A. Charoo, S. H. Ansari and J. Ali, “Pharmaceutical product development:: A quality by design approach,” International Journal of Pharmaceutical Investigation, vol. 6, no. 3, pp. 129-138, 2016.
3. “ASTM D7891-15 Standard Test Method for Shear Testing of Powders Using the Freeman Technology FT4 Powder Rheometer Shear Cell,” ASTM International, 2016.
4. K. Johanson, “Effect of particle shape on unconfined yield strength,” Powder Technology, vol. 194, no. 3, pp. 246-251, 2009.
Acknowledgement
This paper was written by Jennifer Vail PhD and Sarah Cotts, Rheology Product Specialist. Powder Shear experiments run by Terri Chen, PhD.
TA Instruments has been long recognized as an innovator and leader in modulated thermal analysis.
Click here to download the printable version of this application note.